Introduction
The ocean influences weather and climate through air-sea heat and moisture fluxes that affect the stability and vertical motion of the lower atmospheric boundary layer, driving atmospheric circulation anomalies and teleconnections in weather patterns. Moisture originating from the ocean also feeds the hydrological cycle and thus modulates the global distribution of water resources. Disruptions to the hydrological cycle can cause droughts and floods that have enormous societal impacts. In turn, air-sea exchanges of heat, momentum, and mass drive ocean currents, eddies, and turbulent transport between the surface and the ocean interior, setting up the distribution of temperature and salinity that maintains geostrophic currents and regulates biogeochemistry and nutrients in the ocean. The oceans absorb anthropogenic carbon dioxide (CO2), thereby reducing its impact on global atmospheric warming but also causing ocean chemistry changes called ocean acidification. Thus, in an effort to make transformative improvements to Earth system (weather, climate, ocean, and ecosystem) forecasts and CO2 ocean uptake assessments, a new UN Decade of Ocean Sciences for Sustainable Development (2021–2030) program was formed in 2021. This Observing Air-Sea Interactions Strategy program (OASIS; Cronin et al., 2022) links an expanded network of in situ air-sea interaction observations to optimized satellite observations, improved ocean and atmospheric coupling in Earth system models, and improved ocean information for decision-makers. At OASIS’s core is a theory of change: that transformation will come by working together across disciplines and around the world, and by developing a culture of mentorship and partnership.
This theory of change has been key to the success of the NOAA Pacific Marine Environmental Laboratory’s (PMEL’s) Ocean Climate Stations (OCS) project, whose mission is to provide in situ measurements for quantifying air-sea interactions critical to Earth’s energy (heat), water, carbon, and life cycles. PMEL OCS maintains two surface moorings in the North Pacific (Figure 1), one at the Kuroshio Extension Observatory (KEO) in the Northwest Pacific subtropical recirculation gyre and the other at Station Papa in the Northeast Pacific subpolar gyre. Each of these OCS moorings involve multiple partners, were initiated during process studies, and contribute to the global Ocean Sustained Interdisciplinary Time series Environmental Observatory (OceanSITES) network of moorings (http://www.oceansites.org), whose goal is to collect, deliver, and promote the use of long-term observations from fixed sites in alignment with findable, accessible, interoperable, and reusable (FAIR) data principles (Tanhua et al., 2019).
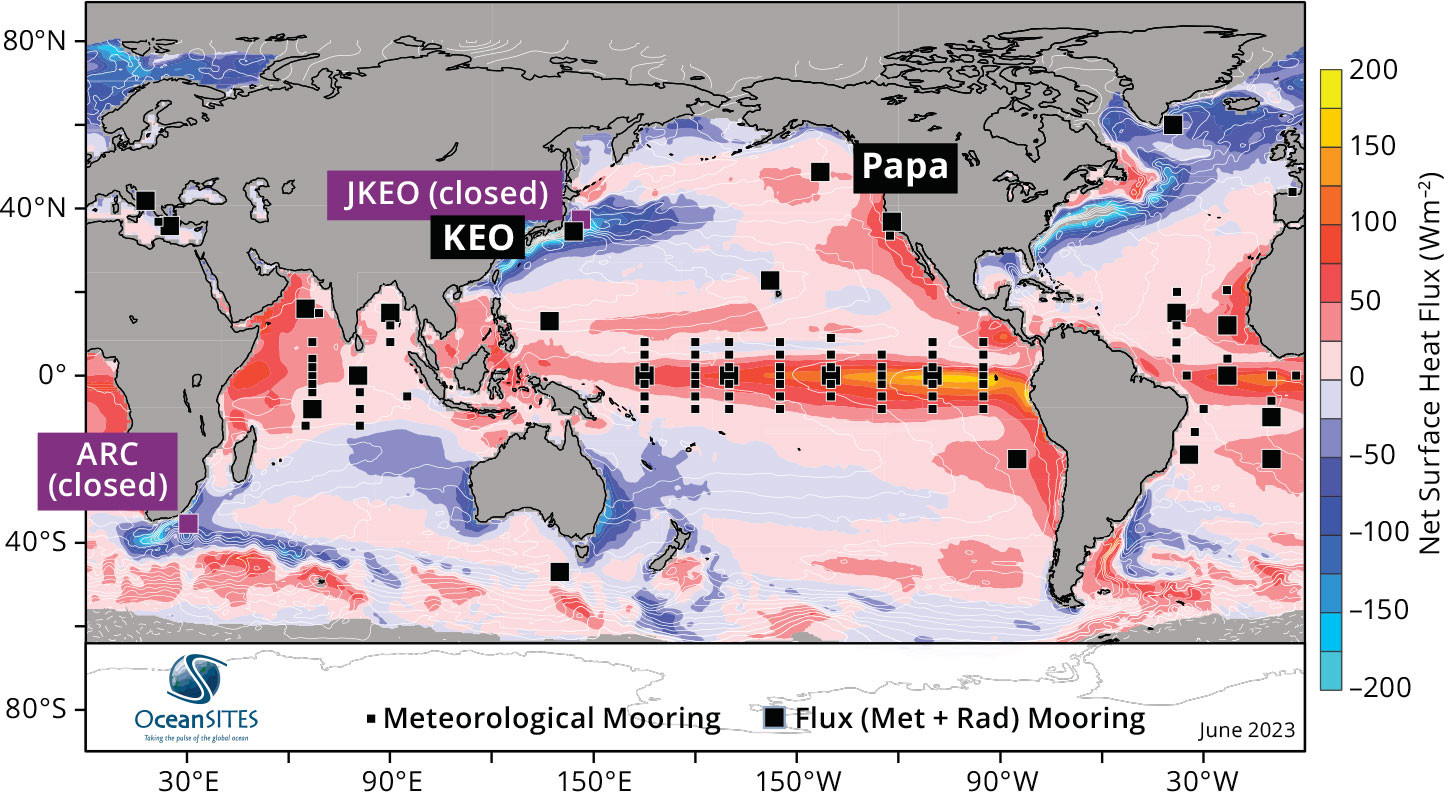
FIGURE 1. The Ocean Sustained Interdisciplinary Time series Environmental Observatory (OceanSITES) network of surface moorings as of June 2023. Locations of moorings at Ocean Climate Stations Kuroshio Extension Observatory (KEO) and Papa, along with the retired Agulhas Return Current (ARC) and JAMSTEC-KEO (JKEO) sites, are shown on a map of currently operational OceanSITES meteorological (small square) and air-sea flux (large square) sites overlaid on the mean net surface heat flux (W m–2) computed from OAFlux-HR and CERES EBAF for 2001–2015. White contours show the climatological sea surface height and can be interpreted as approximate streamlines of geostrophic surface flow. Online supplementary Figure S1 provides a generic map of the active OceanSITES surface mooring sites as of June 2023. Based on Figure 8 from Cronin et al. (2019). > High res figure
|
In this paper, we describe the origin story for OCS and lay out its trajectory through the past two decades and into the future. This retrospective is intended to highlight not only achievements of the project but also some of the challenges faced by OCS and other similar projects. Finally, we will discuss the role the OASIS theory of change has played in the growth of the OCS project.
Ocean Climate Moorings and Fish Aggregate Devices
In 2003, OCS was established as an offshoot of the PMEL Tropical Atmosphere and Ocean (TAO) group at the conclusion of the project entitled “Enhancement of the Tropical Atmosphere and Ocean (TAO) 95°W mooring line for the Eastern Pacific Investigation of Climate (EPIC) process study,” led by principal investigator Meghan Cronin and co-principal investigator Michael McPhaden. As part of the TAO/EPIC project, three additional moorings were added to the easternmost TAO mooring line (95°W): one at 3.5°N to better resolve the equatorial cold tongue front, and two more at 10°N and 12°N to observe the Intertropical Convergence Zone (ITCZ) and Eastern Pacific Warm Pool (Cronin et al. 2006a,b; McPhaden et al., 2008). In addition, to serve the EPIC research community and operational aspects of the array, all 10 moorings were enhanced to measure and telemeter downwelling solar and longwave surface radiation, rain rate, barometric pressure, salinity at seven depths between 1 m and 120 m, and horizontal currents at 10 m. Moorings within the 5°N to 5°S equatorial band were also enhanced with an additional current meter at 40 m. Unfortunately, due to biofouling and pelagic fisheries in the region, the moorings became fish aggregating devices (Dempster and Taquet, 2004). Intense vandalism by the international fishing fleet resulted in many sensor and system failures and a discouraging loss of data and equipment. Ultimately, it was decided to not leave a legacy enhanced 95°W line. Instead, with approval granted by the NOAA Climate Programs Office, at the conclusion of the TAO/EPIC project, the remaining equipment was distributed between the Indian Ocean Research Moored Array for African-Asian-Australian Monsoon Analysis and Prediction (RAMA) led by Michael McPhaden, and a new extratropical site, KEO, led by author Cronin.
Ocean Climate Stations as Research Aggregate Devices
Kuroshio Extension Observatory
The KEO surface mooring was deployed in June 2004 (Figure 2) as part of the two-year National Science Foundation (NSF)-funded Kuroshio Extension System Study (KESS), a process study designed to improve understanding of the ocean dynamics affecting the Kuroshio Extension (KE) and its interactions with recirculation gyres to the north and south (Donohue et al., 2008; Jayne et al., 2009). While KEO is south of the strongest KE jet (Figure 1), it is subject to strong eddy currents, waves, and winds associated with frequent winter storms and warm-season tropical cyclones. In this region, current speeds can occasionally exceed 1.5 m s–1, and during typhoons, winds can exceed 35 m s–1. To survive these harsh conditions, the mooring line’s scope was increased, fairings were added to the upper portion of the mooring line, and the buoy buoyancy and anchor weight were both increased. A load cell was added to provide data to fine-tune the engineering mooring design model used for KEO. The wind sensor was replaced with a sonic anemometer that could withstand stronger winds, and duplicate meteorological sensors were added to improve the chances of providing year-long data sets (Lawrence-Slavas et al., 2006). With a full suite of meteorological, oceanic, and biogeochemical sensors, KEO monitors the large air-sea fluxes of heat, moisture, momentum, and carbon dioxide; ocean acidification and upper ocean temperature and salinity (including a mixed layer depth that ranged from ~10 m to nearly 500 m below the surface); and near-surface currents and shears (Cronin et al., 2013).
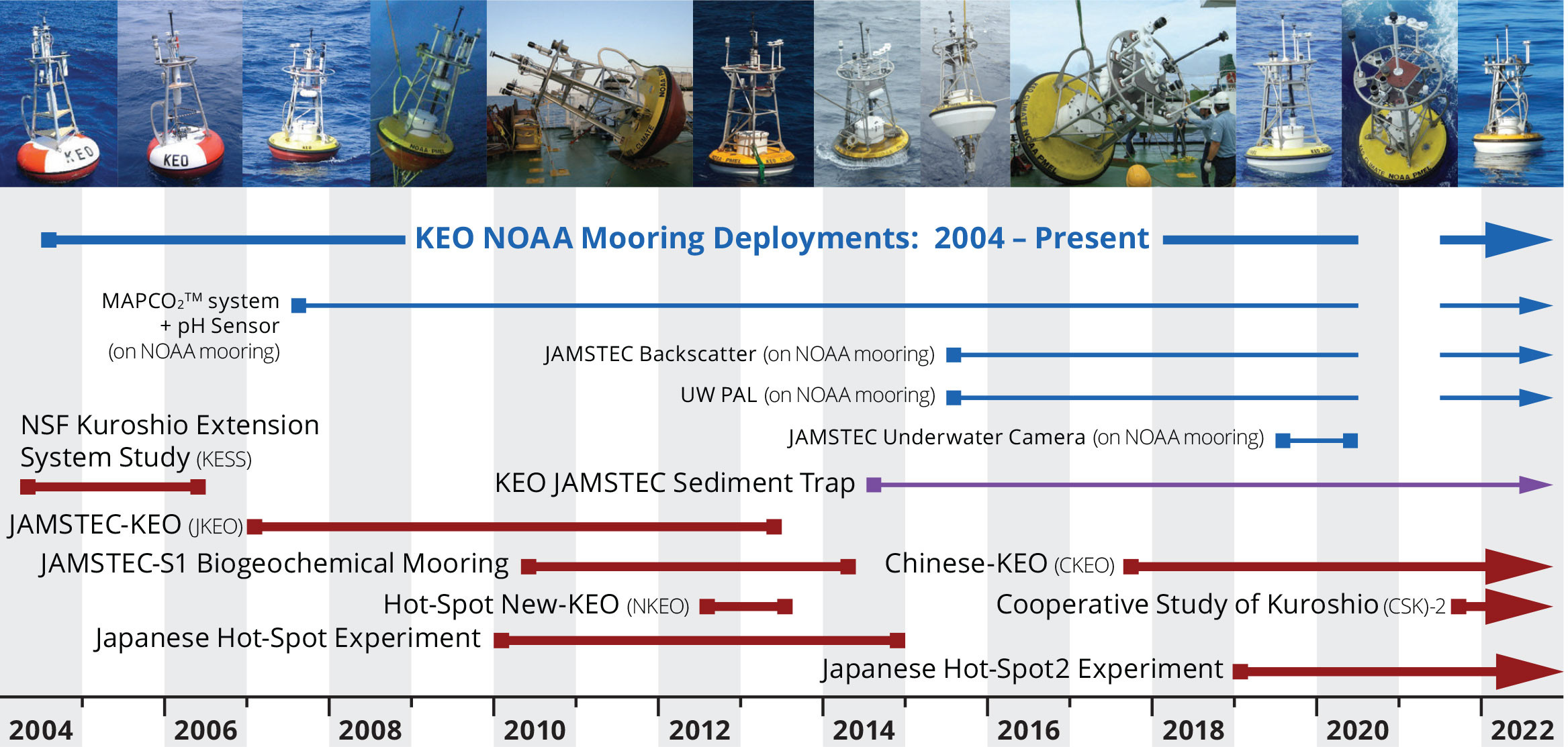
FIGURE 2. Timeline of the Kuroshio Extension Observatory (KEO) and observations in the KEO region. NSF = National Science Foundation. JAMSTEC = Japan Agency for Marine-Earth Science and Technology. UW PAL = University of Washington Passive Acoustic Listener. > High res figure
|
Building upon the long-term partnership between NOAA PMEL and the Japan Agency for Marine-Earth Science and Technology (JAMSTEC), in 2005, a Japanese KEO (J-KEO) was established north of the KE (Figures 1 and 2). The two KEO moorings, north and south of the KE, enabled frontal studies, including research into the impacts of the KE front on air-sea fluxes (Konda et al., 2010) and weather patterns (Tomita et al., 2021a,b). These frontal studies were expanded as part of the Japanese Hot-Spot experiment (2010–2014) to include intensive observations and model studies of the atmospheric response to the very large wintertime air-sea heat fluxes (“hot spots”) found in western boundary current regions (Nakamura et al., 2015). While the J-KEO site (Figure 1) was discontinued in 2013, in 2014 (Figure 2) JAMSTEC deployed a sediment trap mooring next to the NOAA surface mooring at KEO (Honda et al., 2018). Likewise, the follow-on Japanese Hot-Spot-2 experiment (2019–2023) has focused on the multidisciplinary impacts of these large air-sea fluxes, including those that affect the ocean’s biogeochemistry. China has now deployed a set of C-KEO moorings north and south of the KE jet, and Korea and other nations are making coordinated intensive observations in the Kuroshio and its adjacent seas. The NOAA OCS surface mooring at KEO is regularly enhanced with sensors from partners in Japan and at the University of Washington and welcomes collaborative work with other partners. Hearkening back to a golden era of international collaboration from 1965 to 1979, spurred by the Cooperative Studies of the Kuroshio and Its Adjacent Seas (CSK), in 2021, a Second CSK (CSK-2) was formed that includes roughly a dozen projects from member states, including one from NOAA (USA) and JAMSTEC (Japan) for the KEO station.
KEO data, like all OCS data, are publicly available and used widely. Here, we highlight just a few of the many scientific studies using KEO data. During the KESS experiment, the KE jet switched from a quasi-stable to an unstable state (Donohue et al., 2008). The KEO surface mooring was critical for monitoring the interannual variations in Subtropical Mode Water formation within the recirculation gyre south of the KE (Rainville et al., 2014). Cronin et al. (2015) show that the very deep wintertime surface mixed layer at KEO leads to an effective heat capacity that is much larger than it is on the cold side of the KE jet. Consequently, sea surface temperature (SST) on the warm side of the KE jet is much less sensitive to air-sea heat fluxes than it is on the cold side. Indeed, even though surface ocean heat loss is larger over the warmer waters south of the KE jet during winter, SST cools more rapidly on the north side of the KE, leading to a strengthening of the SST front (Tozuka et al., 2017). Further analyses found that throughout the global ocean, but particularly in regions of strong baroclinic currents, a cross-front gradient in the mixed layer depth can play a role in strengthening and weakening the SST front (Ohishi et al., 2017; Tozuka et al., 2017, 2018, 2023). Because the air-sea heat fluxes depend in part upon the strength of the SST front, poor representation of mixed layer depth could lead to negative rather than positive feedbacks between the SST front and ocean surface heat loss. This could partially explain why coarse resolution coupled models, which typically have poor mixed layer physics, have an unrealistic atmospheric response to ocean forcing in western boundary current regions (Tozuka et al., 2017).
KEO data have been used to analyze the ocean’s response to an extratropical transition of a class 1 typhoon (Bond et al., 2011; Wada et al., 2013). Using surface mooring and subsurface sediment trap data, Honda et al. (2018) show that the typhoons did not cause a significant export of carbon to depth; however, cold core eddies did. The anomalously shallow pycnocline at the center of cold core eddies brings nutrients into the euphotic zone, leading to subsurface blooms that are followed by sediment accumulations in the deep ocean. This explains the mystery of how this otherwise oligotrophic region can support a large biogenic flux.
KEO is one of the few extratropical sites within the global network of OceanSITES stations and is unique in its setting within a western boundary current system (Figure 1). Because of the large dynamic range of environmental conditions and the multiple co-located variables observed at KEO, this site has become a favorite testbed for validating satellite products (e.g., Tomita et al., 2019; Takeyama et al., 2019; Koizumi et al., 2020) and for assessing numerical weather prediction models (Kubota et al., 2008; Zhang et al., 2016). While the World Meteorological Organization numbers for the KEO and Papa moorings identify their products as reference data that should be withheld from assimilation, it is unclear whether that actually happens. However, even if ingested by data assimilation software, these data remain useful for intercomparisons, because if the buoy observations differ significantly from the modeled state, they are rejected and thus are independent of the product. Efforts to determine whether or not these data are assimilated in the numerical weather prediction reanalysis products are strongly encouraged and require close collaboration between observationalists and modelers. In addition to identifying mean biases and scatter in differences, the high resolution, co-located time series can often be used to diagnose the cause of the error by identifying discrepancies in, for example, the variables (e.g., humidity, winds, SST) or the phenomena (e.g., gustiness, onshore or offshore winds, clouds, fronts) or the bulk flux parameterization. Such information helps model developers improve model physics and products.
Ocean Station Papa
OCS formed in earnest in 2007 when the PMEL OCS surface mooring was deployed at Station Papa (50°N, 145°W) through an NSF-funded carbon cycle experiment (Emerson et al., 2011) and with ship time provided by the Canadian Department of Fisheries and Oceans (DFO) Line P program (Freeland, 2007). Station Papa (Figure 3), located in the Northeast Pacific subpolar gyre, 850 nm west of British Columbia, is one of the oldest ocean time series in the world. From 1949 to 1981, an ocean weather ship was stationed at Papa, and since 1956, regular ship-based hydrographic “Line P” sections between Station Papa and the coast have been made. At the conclusion of the NSF experiment, the NOAA Climate Programs Office (now Global Ocean Monitoring and Observing, or GOMO) continued funding the PMEL OCS surface mooring as a contribution to the OceanSITES network of long-term reference time series. As with KEO, the PMEL OCS surface mooring at Papa monitors air-sea fluxes of heat, moisture, momentum, and carbon dioxide; surface and subsurface temperature and salinity; and upper ocean currents. In addition, the Papa mooring was the first deep ocean platform to establish a dual-parameter autonomous time series (seawater pCO2 and pH) for monitoring ocean acidification (Sutton et al., 2016). This package was later added to the KEO mooring and other open ocean and coastal moorings supported by GOMO and the NOAA Ocean Acidification Program (Sutton and Sabine, 2023, in this issue).
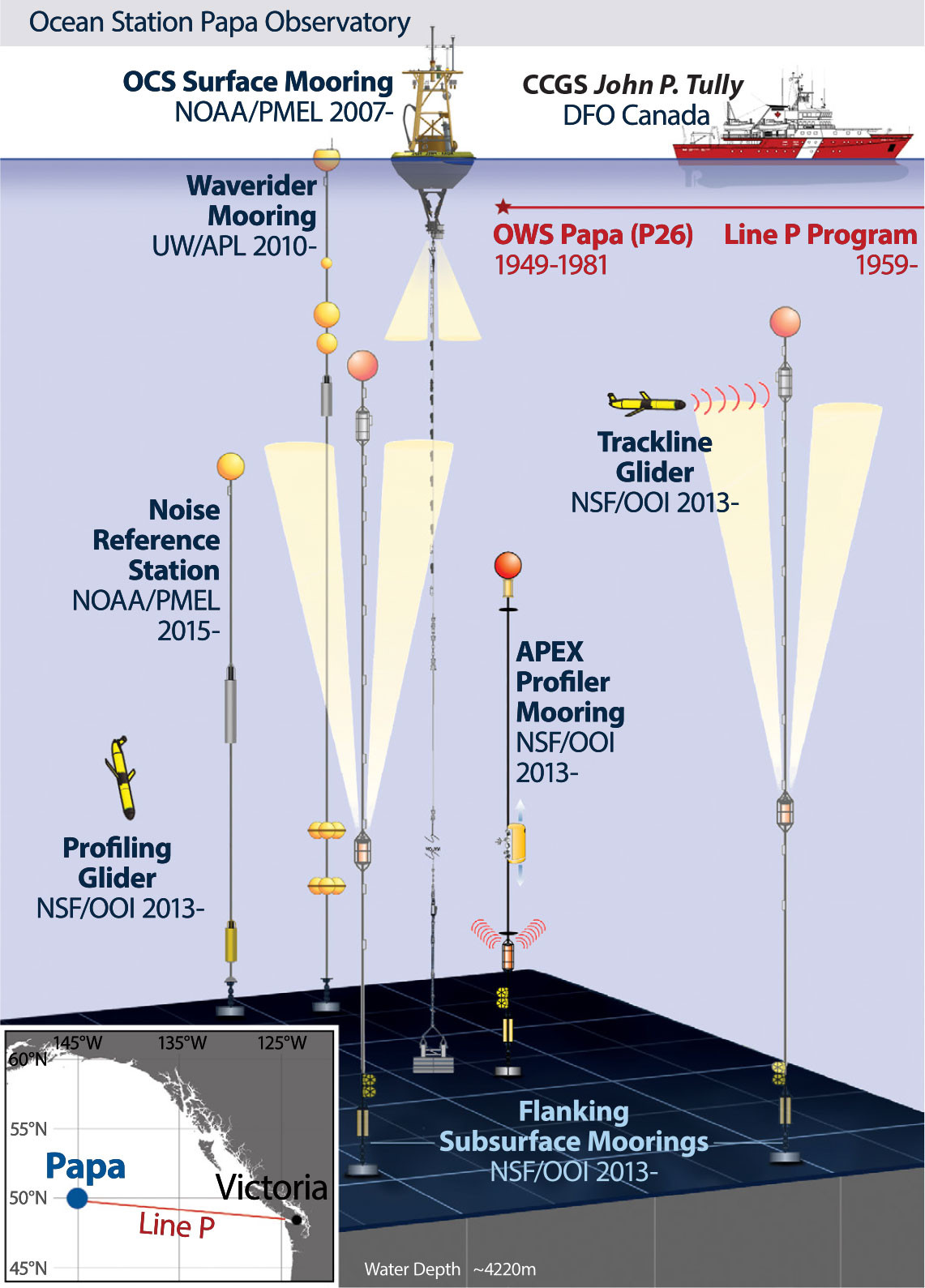
FIGURE 3. Ocean Station Papa observatory as of June 2023. OCS = Ocean Climate Stations. NSF/OOI = National Science Foundation/Ocean Observatory Initiative. UW/APL = University of Washington/Applied Physics Laboratory. OWS Papa = Ocean Weather Ship Papa. > High res figure
|
Just as the Line P program with its regular cruises to Station Papa was a major attraction for the OCS surface mooring, the ongoing OCS observations have attracted many new partners. In 2010, with funding from NSF, the first deep ocean waverider mooring was deployed by the University of Washington’s Applied Physics Laboratory (UW/APL) at Station Papa. Good agreement between the bulk wind stress measured by the OCS surface mooring and equilibrated wind stress estimated from the wave spectrum (Thomson et al., 2013) demonstrated a new methodology for computing wind stress from wave measurements that is now being used commercially by companies such as SOFAR Inc. (Voermans et al., 2020). The UW/APL wave measurements are now being combined with the meteorological observations and backscatter intensity measurements from a downward looking acoustic Doppler current profiler on the PMEL OCS surface mooring to investigate the connections between wind forcing, surface wave breaking, bubble production, gas exchange, and ambient underwater sound, with a particular focus on high sea states (Zappa et al., 2007; Liang et al., 2017).
In 2013, the NSF-funded Ocean Observatories Initiative enhanced Station Papa (Figure 3) with subsurface moorings and underwater gliders to make it one of four (now two) global nodes (Trowbridge et al., 2019), relying upon the PMEL OCS surface mooring to serve as its “central mooring.” In 2015, NOAA PMEL deployed a noise reference station subsurface mooring at Station Papa that monitors the soundscape, from blue (Balaenoptera musculus), fin (Balaenoptera physalus), and other vocal baleen and odontocete cetacean species to human-made sound associated with ship traffic (Pearson et al., 2023). Figure 3 shows the full Ocean Station Papa observatory.
As with KEO, we highlight only a couple of the many scientific studies that have taken advantage of PMEL OCS Station Papa data. Using air-sea heat flux, ocean mixed layer depth, temperature, and current measurements from the OCS surface mooring, and SST gradients from a satellite blended product, Cronin et al. (2015) closed the surface mixed layer heat budget and estimated diffusivity across the base of the mixed layer from the budget residual. Its annual climatology was then used in the dissolved inorganic carbon and total alkalinity budgets computed from the mooring observations and climatological fields to estimate the net community production and calcium carbonate production components of the biological carbon pump (Fassbender et al., 2016). A similar study was later done at KEO (Fassbender et al., 2017). Among the most profound research originating at Station Papa was the observation of substantial marine heatwaves in 2013–2016 and 2019, with peak seawater temperature anomalies in February 2014 exceeding +2.5°C in the upper 100 m of the northeastern Pacific. Bond et al. (2015) referred to the 2013 marine heatwave as “the Blob,” a moniker that has successfully communicated the extreme nature of this ocean anomaly to public audiences.
Challenges
Harsh open-ocean environments, biofouling, and vandalism occasionally lead to sensor and system failures or broken mooring lines, with resultant time series gaps. Through careful engineering and duplicate systems, these failures are minimized, although they remain an ongoing risk. Obtaining ship time for the annual turnaround cruises, and unanticipated breaks, has been one of the greatest challenges faced by the PMEL OCS project. NOAA, PMEL, and the entire OCS team are deeply grateful to partners in Japan, Canada, South Africa, France, and the United States who have provided ship time both planned and unplanned.
Despite the involvement of many active partnerships, a broken mooring line resulted in the discontinuation of a new station in the Agulhas Return Current intended for the OceanSITES network. Like KEO and Papa, the Agulhas Return Current mooring (Figure 1) was deployed as part of an NSF-funded process study, the Agulhas Current Time Series Experiment (Beal et al., 2015). Ship time for its deployment was provided by the Agulhas–Somali Current Large Marine Ecosystem program. Unfortunately, after a couple of months, the mooring broke. Although the drifting buoy and all its sensors were recovered by R/V Marion Dufresne, which was en route to Antarctica, sustained funding from NOAA GOMO could not be secured, and the mooring was not redeployed.
Deep ocean moorings are difficult to scale and require mariners with highly specialized at-sea skills. Facing a 10% cut or year after year of flat funding, what part of a mooring can be eliminated? Sensors? Satellite telemetry? Calibrations? Ship time? Personnel? OCS surface mooring managers have tried to balance the needs for advanced instrumentation and redundancy with the realities of operating within a limited budget. Partner groups, since inception, have supported and funded various systems, such as the CO2 and ocean acidification suite of sensors. In FY22, the core OCS cost of operating both KEO and Papa, not including ship time or partner systems, was $825K. Until recently, ship time for Papa was provided through the Line P program. The Papa mooring observatory (Figure 3), however, has grown beyond the capacity of CCGS Tully. Now the three mooring groups work together, purchasing sea days on each other’s cruises to complete the work in the most efficient way. At the start of the OCS project, nearly two decades ago, the OCS project funded 23 people, but each for only a few weeks at a time. The project still outsources some work to PMEL’s TAO group (now called Global Tropical Moored Buoy Array, or GTMBA) and PMEL’s Engineering Development Division, and routinely brings a second or third cruise participant from other groups. Most of the technical work, however, is performed by just two highly experienced ocean professionals (authors Patrick Berk and Nathan Anderson), with scientific leadership provided by the principal investigator, author Cronin, and the co-principal investigator, author Zhang, who is supported for three to six months through the OCS budget. The minimal co-principal investigator support and lack of support for early career technicians, scientists, postdocs, and students is a lost opportunity for incubating talent and serving the scientific community. As OCS looks toward the future and the need grows to identify the next generation to carry the project forward, succession planning will become increasingly important.
The Future
Although surface moorings can sometimes be fish aggregating devices and subject to vandalism, these platforms are uniquely suited for creating long time series of many essential ocean variables, and they provide extraordinary opportunities for research. Indeed, we have shown through the stories of KEO and Station Papa how regularly scheduled research cruises, such as the DFO Line P program, and long-term surface moorings can act as research aggregating devices, where a core infrastructure is leveraged by partners for multidisciplinary observations serving a wide range of users well beyond the original mission. These co-located enhancements add dimension that can foster new insights into the complexities of the Earth system.
As the climate changes due to anthropogenic greenhouse gas emissions, the long time series will be increasingly important for understanding impacts not only on ocean heat content but also on ocean chemistry, the ocean-atmosphere hydrological cycle, and the marine ecosystem. Disturbing trends in these processes that are emerging at both KEO and Papa (Sutton et al., 2017) require action. Looking forward, the OCS project at NOAA PMEL will continue to develop low-carbon footprint oceanographic observing methods. Since 2016, OCS has expanded its scope to include the use of uncrewed surface vehicles (USVs) to sample the ocean. “Saildrones” are USVs powered by wind for propulsion and solar energy for electronics. Transforming a sailing drone into an ocean observing platform was a major PMEL effort in partnership with Saildrone Inc. (Meinig et al., 2019). In order to develop an adaptive sampling strategy for integrating USVs into the Tropical Pacific Observing System (TPOS), by spring 2023, OCS had conducted five USV missions to the equatorial Pacific (Figure 4; Zhang et al., 2019; Wills et al., 2021). The USV suite of surface sensors is similar to that on OCS buoys, with the capability to monitor air-sea heat, momentum, and carbon fluxes, as well as upper ocean currents. In our upcoming 2023 mission from Hawai‘i to 0°, 155°W, one saildrone will carry an echo sounder for observing biomass distribution from a range of trophic levels, thus adding a fisheries dimension to the integrated TPOS observations.
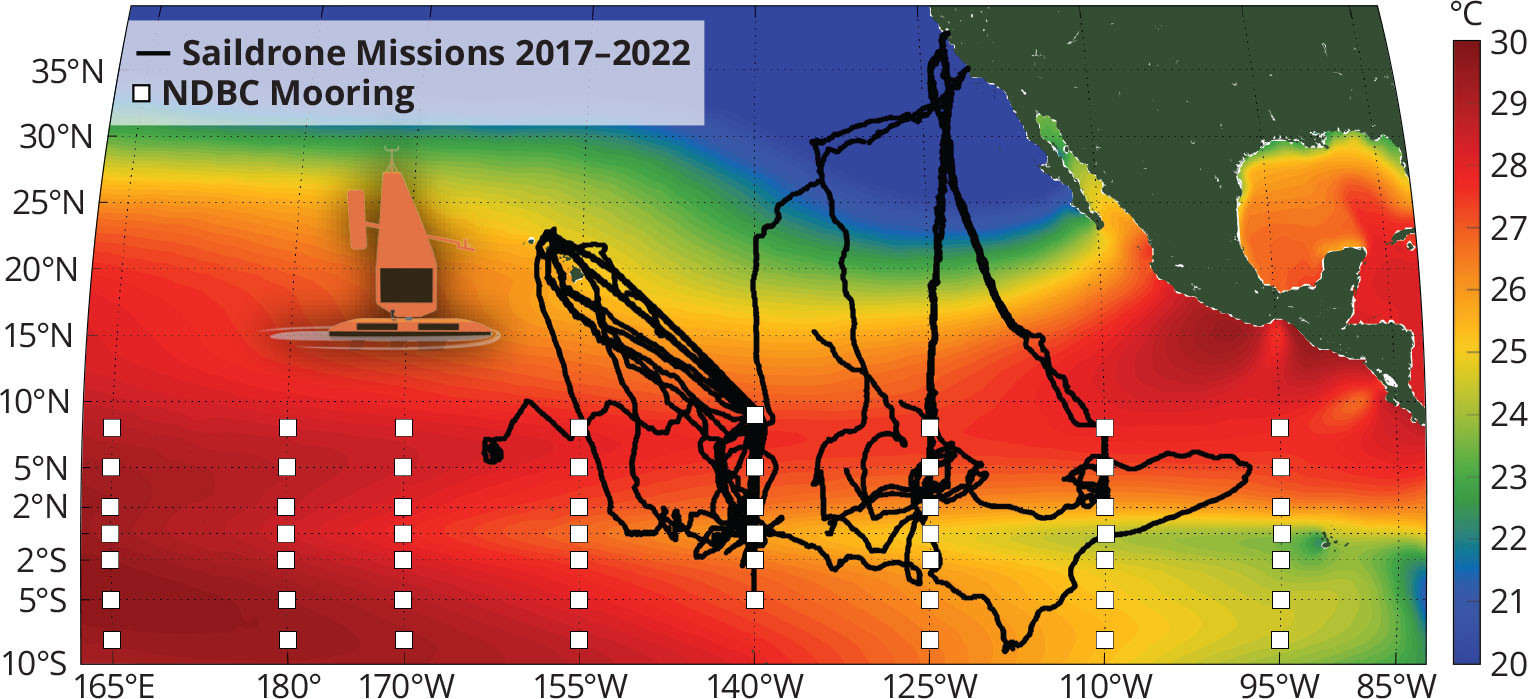
FIGURE 4. Tracklines of the 2017, 2018, 2019, 2021, and 2022 Saildrone Tropical Pacific Observing System (TPOS) missions. The National Data Buoy Center (NDBC) surface mooring array (white squares) forms the backbone of the TPOS. > High res figure
|
Since the early 1980s, it has been recognized that air-sea interactions in the tropical Pacific associated with El Niño can cause worldwide patterns of anomalous drought and flooding on interannual timescales. The TPOS mooring array was designed in the late 1980s to monitor key elements of these air-sea interactions. Newer technologies, however, such as Argo floats (Roemmich et al., 2009) and satellites have greatly expanded the TPOS capability, while ship time has become more expensive. Thus, from 2014 to 2020, an international review of the integrated TPOS, referred to as TPOS-2020, was carried out (Cravatte et al., 2016; Kessler et al., 2019). Lessons learned through OCS participation in this review process are now being applied globally through the OASIS UN Decade of Ocean Science for Sustainable Development program. Likewise, lessons learned through 20 years of working with partners on the OCS project have led to the formation of the OASIS Theory of Change: that transformation and opportunities will come by working together across disciplines and around the world, and by developing a culture of mentorship and partnership.
Acknowledgments
All OCS data are publicly available from https://www.pmel.noaa.gov/ocs/ and through the http://www.oceansites.org/ data portal. Partner data are publicly available through their own websites, which can be accessed from https://www.pmel.noaa.gov/ocs/partners-data. The authors would like to thank Marie Robert (DFO Institute of Ocean Sciences) and the DFO Line P program for providing ship time to service the NOAA Papa mooring for many years; Steve Emerson (University of Washington) for providing initial funding and vision for Papa; Bo Qiu (University of Hawai‘i), Nelson Hogg (formerly of the Woods Hole Oceanographic Institution), Steve Jayne (Woods Hole Oceanographic Institution), and other KESS PIs without whom KEO would not have gotten started; Hiroshi Ichikawa (formerly of JAMSTEC), our original JAMSTEC partner; Hisashi Nakamura (University of Tokyo), Masami Nonaka (JAMSTEC), and other HotSpot PIs; former OCS co-principal investigator Nick Bond (University of Washington); Jim Edson (Woods Hole Oceanographic Institution) and the NSF OOI program; Robert Dziak (NOAA PMEL) and the Ocean Noise Reference Station program; our saildrone fisheries partner Réka Domokos (NMFS/PIFSC); our NOAA/NCEP modeling partners Arun Kumar, Jieshun Zhu, and Avichal Mehra; and critically, Robert Kamphaus (formerly of NOAA Corps) and Andy Shepherd, who together with Chris Meinig (both formerly of NOAA PMEL), helped create the spinoff OCS project. We are also extremely grateful for all the technical support received from the GTMBA and engineering groups and to former OCS technical leads Patrick A’Hearn, Keith Ronnholm, and Jennifer Keene. Funding for KEO and Papa from NOAA Global Ocean Monitoring and Observing is gratefully acknowledged. Funding for the TPOS Saildrone missions has been provided by NOAA Global Ocean Monitoring and Observing, NOAA Office of Marine and Aviation Operations, NOAA Uncrewed Systems Transition Office, and NOAA National Oceanographic Partnership Program. This is PMEL contribution 5482.