Origins of EcoFOCI
In the spring of 1980, the echosounder of a NOAA ship returning from the Bering Sea detected a massive fish signal in Shelikof Strait, Gulf of Alaska (GOA). This was verified in 1981 by a directed survey (see echogram image on title page)—a finding that would expand NOAA ecosystem and fisheries studies in Alaskan waters.
Prior to this survey, physical oceanographers from the NOAA Pacific Marine Environmental Laboratory (PMEL) and biologists from the National Marine Fisheries Service (NMFS) conducted joint studies through an informal partnership that began shortly after the formation of PMEL in 1973, when intense field programs were launched in the GOA and the Bering Sea. The Shelikof Strait echogram set in motion a series of events that resulted in a formal partnership between two laboratories that has become the gold standard of collaboration within NOAA line offices.
Subsequent to the echogram, sampling in Shelikof Strait revealed spawning walleye pollock (Theragra chalcogramma, later reclassified Gadus chalcogrammus), and large numbers of eggs (Kendall et al., 1987). In 1982, PMEL’s director, Eddie Bernard, met with Bill Aron, director of the Northwest and Alaska Fisheries Science Center (NWAFSC, later split into two Science Centers, AFSC and NWFSC) to discuss common research opportunities. Aron and Bernard forwarded an initiative to their respective headquarters, and staff at NOAA Fisheries and NOAA Research—having never previously received a joint initiative—liked the idea. NOAA Administrator Anthony Calio learned about the joint proposal, visited Seattle, and in 1984 the initiative was funded. James Schumacher (PMEL) and Arthur Kendall Jr. (AFSC) were chosen as the lead scientists of the new joint program—Fisheries-Oceanography Coordinated Investigations (FOCI, initially Fisheries Oceanography Experiment, FOX; Wilson et al., 1986)—with a goal of understanding recruitment mechanisms and variations in year-class strength of commercially exploited fish in Alaskan waters. Walleye pollock in Shelikof Strait was the initial focus (Reed et al., 1988).
In the following decades, the breadth and depth of research into ecosystem dynamics expanded with observations, long-term monitoring, laboratory experiments, and modeling across four of Alaska’s five large marine ecosystems—GOA, Aleutian Arc, Bering Sea, and Chukchi Sea (Figure 1). This research was enabled through a multitude of external grants from various sources, including the North Pacific Research Board (NPRB), the National Science Foundation (NSF), and the Bureau of Ocean Energy Management, and partnerships with other AFSC divisions and NOAA Line Offices, cooperative institutes at the University of Washington and University of Alaska Fairbanks, and academic and international partners. EcoFOCI was a partner in programs such as GLOBal Ocean ECosystems Dynamics (GLOBEC), the first integrated ecosystem study of the Aleutian Islands, and NPRB’s Gulf of Alaska Integrated Ecosystem Research Program. In 2004, an initiative called North Pacific Climate Regimes and Ecosystem Productivity was funded, and FOCI transitioned into EcoFOCI (see Stabeno et al., 2023, in this issue). We highlight here a few contributions regarding the GOA that are among the hundreds of publications produced across the history of EcoFOCI. Other articles in this issue discuss EcoFOCI’s modeling approaches (Hermann et al., 2023) and climate impacts in the US Arctic (Stabeno et al., 2023).
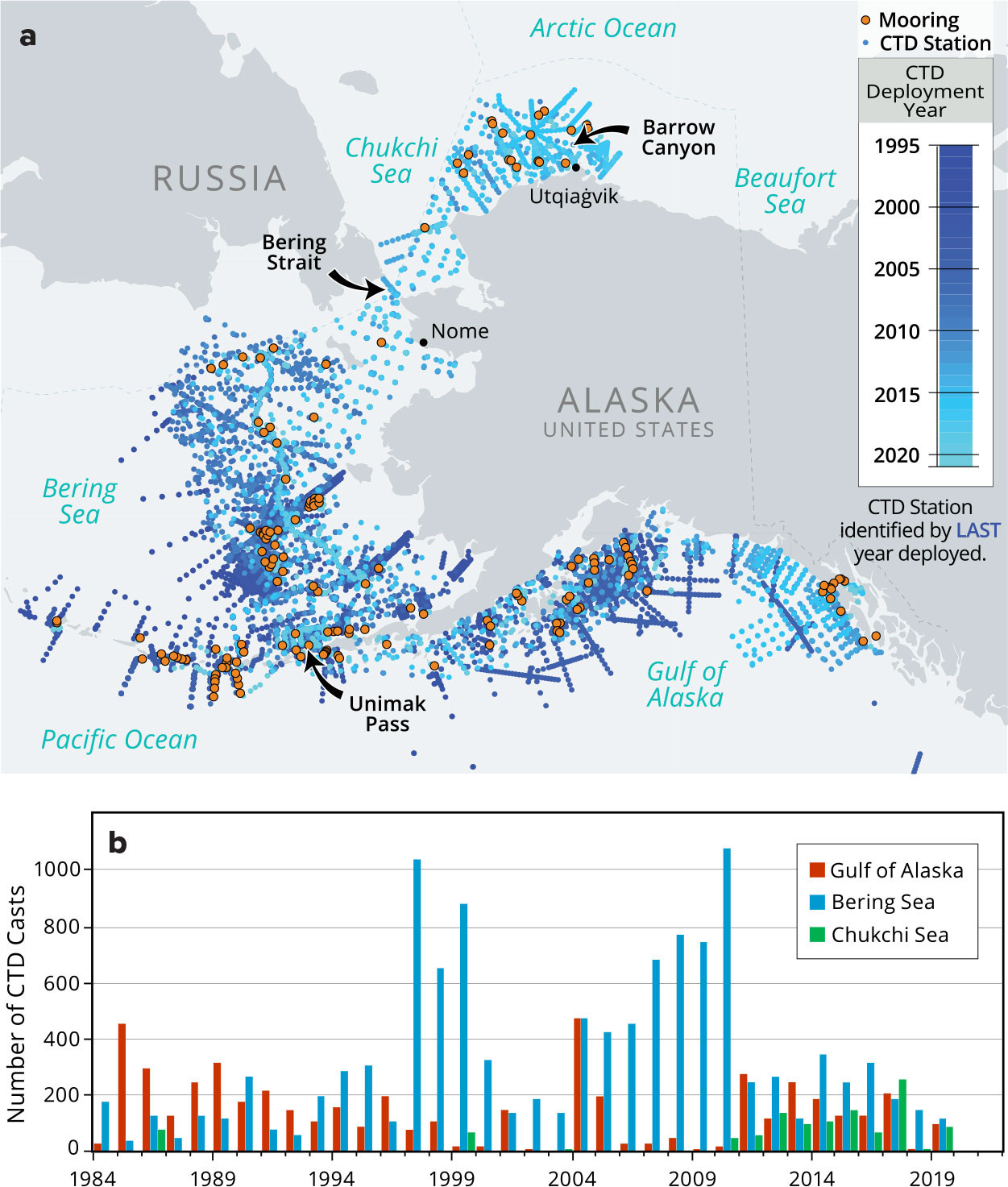
FIGURE 1. (a) Map of Alaskan waters showing the location of Ecosystems & Fisheries-Oceanography Coordinated Investigations (EcoFOCI) moorings (orange dots) and CTD casts (blue dots, colored by year). Adapted from Tabisola et al. (2021) (b) The histogram displays the number of CTD profiles collected on EcoFOCI and collaborative cruises. > High res figure
|
Pollock Recruitment in Shelikof Strait
FOCI’s early emphasis was on recruitment of Shelikof Strait pollock stock that can exhibit order-of-magnitude variability from year to year. Shelikof Strait, located between the Alaskan Peninsula and Kodiak Island, is ~190 km long and 35–60 km wide (Figure 2). To the northeast, most of the Alaska Coastal Current (ACC, Figure 3) flows down Shelikof Strait (Stabeno et al., 1995). Upon exiting the strait, it enters the Shelikof sea valley where it bifurcates. Approximately one-third of the ACC flows between Sutwik Island and the Shumagin Islands, eventually entering the Bering Sea through the eastern Aleutian Passes, while the remainder flows down the Shelikof sea valley to the slope (Stabeno et al., 2016a). Baroclinic instability within Shelikof Strait results in small-scale (10–20 km) eddies occurring within the sea valley (Schumacher and Reed, 1980). The sea valley has estuarine-like circulation with inflow of colder, nutrient-rich slope water near the bottom and on the eastern side.
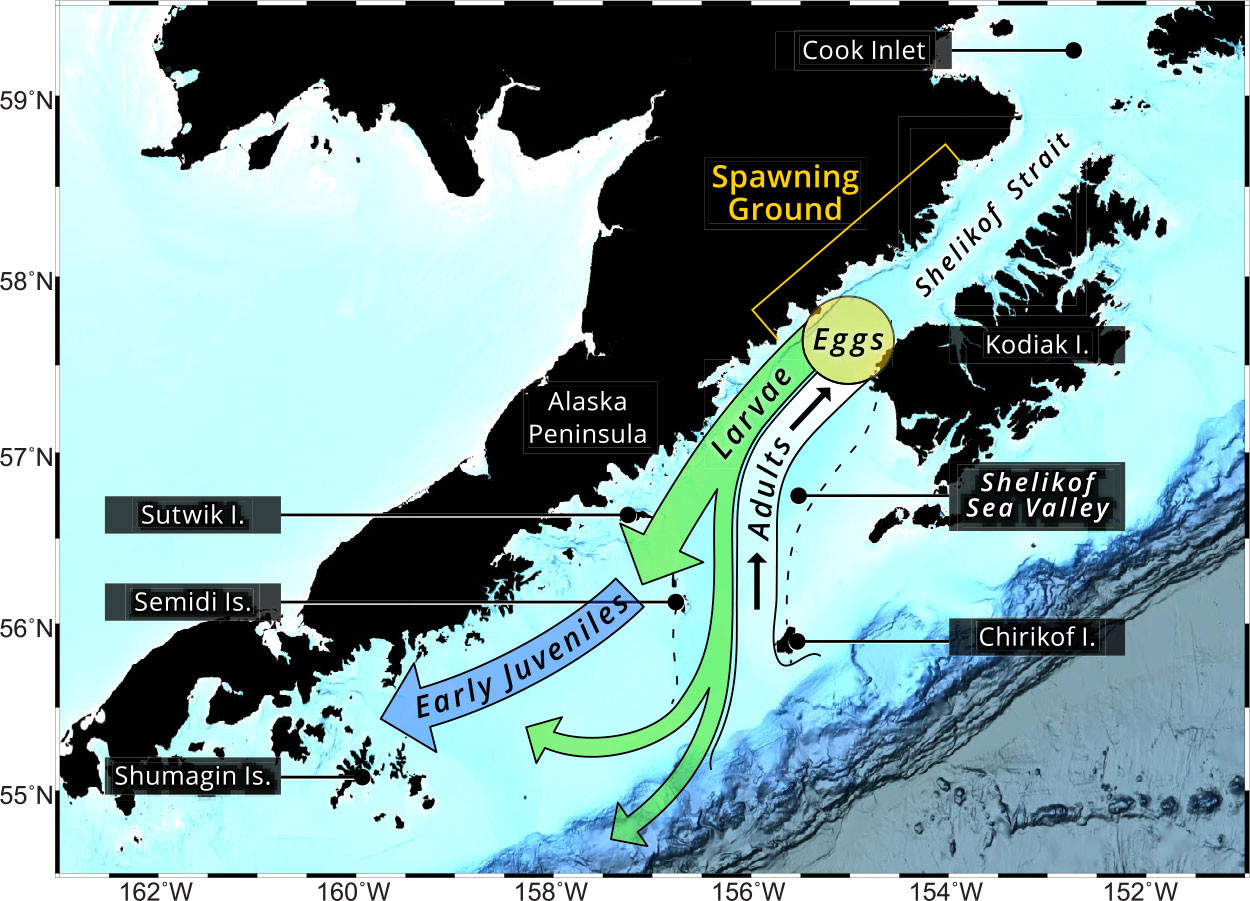
FIGURE 2. Early life history of walleye pollock in the Shelikof Strait region. Black arrows indicate the spawning migration of adults, and the movement of larval (green arrows) and juvenile (blue arrow) pollock. Adapted from Kendall et al. (1996). > High res figure
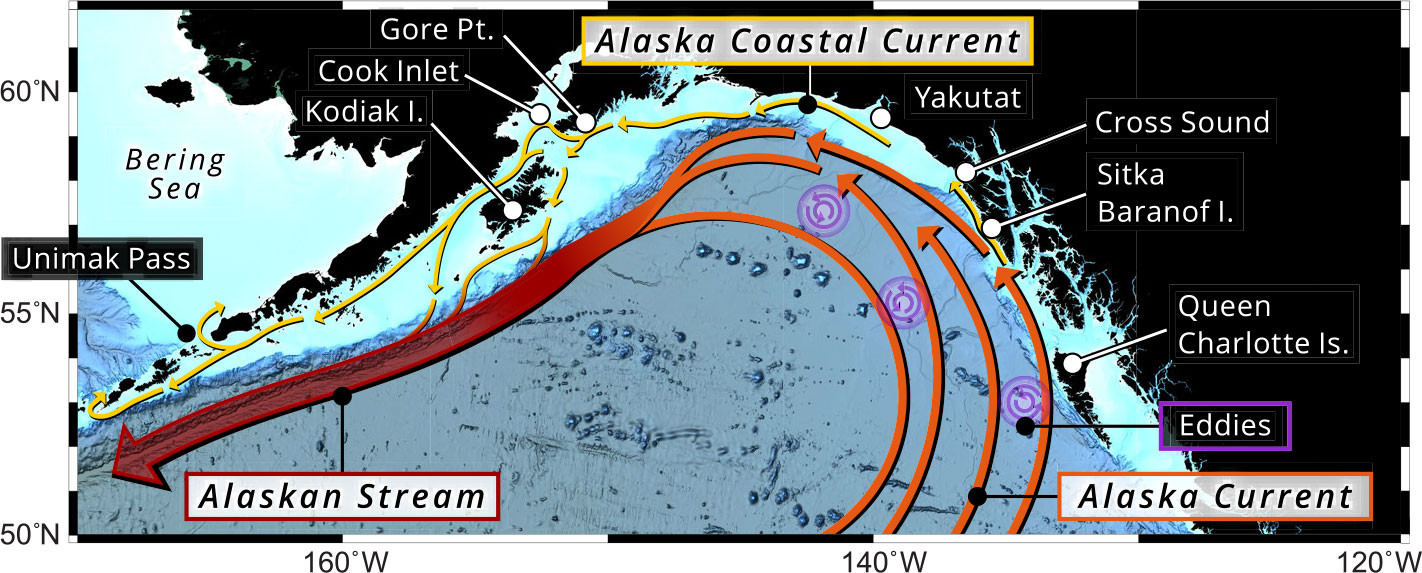
FIGURE 3. Sketch of major currents in the Gulf of Alaska. After Reed and Schumacher (1986). > High res figure
|
|
Following these patterns of flow, adult pollock enter the sea valley to spawn within Shelikof Strait (Figure 2). Larvae hatch after two to three weeks and surface with sufficient swimming ability to maintain larval patches (Schumacher et al., 1993). If retained in an eddy, larvae are less likely to be transported offshore into the Alaska Stream and may remain in the nursery ground near the Shumagin Islands. Biophysical models suggest that transport to the coastal area between the Semidi and Shumagin Islands before mid to late May would not be advantageous due to the lack of prey (e.g., copepod nauplii) in that region early in the year (Hinkley et al., 2009). In the late 1990s, factors determining pollock recruitment switched from environmental control of larval survival (bottom-up control) to predation on juveniles (top-down control) (Bailey, 2000), although currents still influence the geographic distribution and habitat suitability of juveniles.
Physical Oceanography
Under EcoFOCI, the breadth and scope of observations in the GOA now extend from Southeast Alaska to the western Aleutian Passes (Figure 1) in order to resolve the physical dynamics of the region (Stabeno et al., 2004, 2016a, 2016b) that underpin numerous ecosystem studies. Mean flow on the GOA shelf is dominated by the ACC, which is largely continuous over the ~3,000 km coastline from the southern tip of Baranof Island to Samalga Pass in the Aleutian Islands (Figure 3). The ACC is forced by strong cyclonic winds, and its baroclinic structure is controlled by freshwater runoff from rivers and glaciers along the mountainous Alaskan coastline. Along the path of the ACC, enhanced mixing and cross-shelf exchange at various locations bring nutrients into the surface waters in this downwelling-dominated system (Stabeno et al., 2016b; Mordy et al., 2019), contributing to the high productivity of these coastal waters. Spatially, the highest transport in the ACC occurs at Gore Point (~1.4 Sv [Sv =106 m3 s–1]; Stabeno et al., 2016a), with weaker flow in southeast Alaskan waters and to the west.
Eddies are ubiquitous features throughout the GOA and are important for aggregating plankton species (Kroeger et al., 2021). Both the Shelikof sea valley eddies and the larger shelf-break eddies are correlated with high chlorophyll concentrations (Ladd, 2007). In the southeastern GOA, most of the larger basin eddies (~200 km diameter) form at three primary sites: Queen Charlotte Islands, Sitka, and Yakutat. In addition to mesoscale basin eddies, the steep topography lining Cross Sound can funnel the atmospheric flow into strong gap winds that influence the coastal ocean up to 200 km offshore (Ladd and Cheng, 2016). Around Kodiak Island, gap winds influence chlorophyll levels, temperatures, and larval retention by modifying the ACC, driving Ekman pumping and increasing local mixing (Bond and Stabeno, 1998; Ladd et al., 2016). The eddies and gap wind events magnify basin-shelf exchange with meanders and filaments that extend far offshore (see NASA image on title page).
Off the shelf, basin circulation is dominated by the Subarctic Gyre, which tends to be stronger during positive phases of the Pacific Decadal Oscillation (PDO; Hristova et al., 2019). The Alaska Current is the eastern boundary current of this gyre. Off Kodiak Island, the circulation narrows to form the Alaskan Stream, a narrow (~60 km), deep (>3,000 m), high-speed western boundary current with annual mean transport of ~20 Sv (Stabeno and Hristova, 2014). Approximately half of the Alaskan Stream enters the Bering Sea through the deeper Aleutian Passes, particularly Amukta Pass, Amchitka Pass, and Near Strait (Figure 4; Reed and Stabeno, 1994; Stabeno et al., 2005b; Stabeno and Hristova, 2014), transporting heat and salt (including nutrients) into the Bering Sea and providing a pathway for marine species.
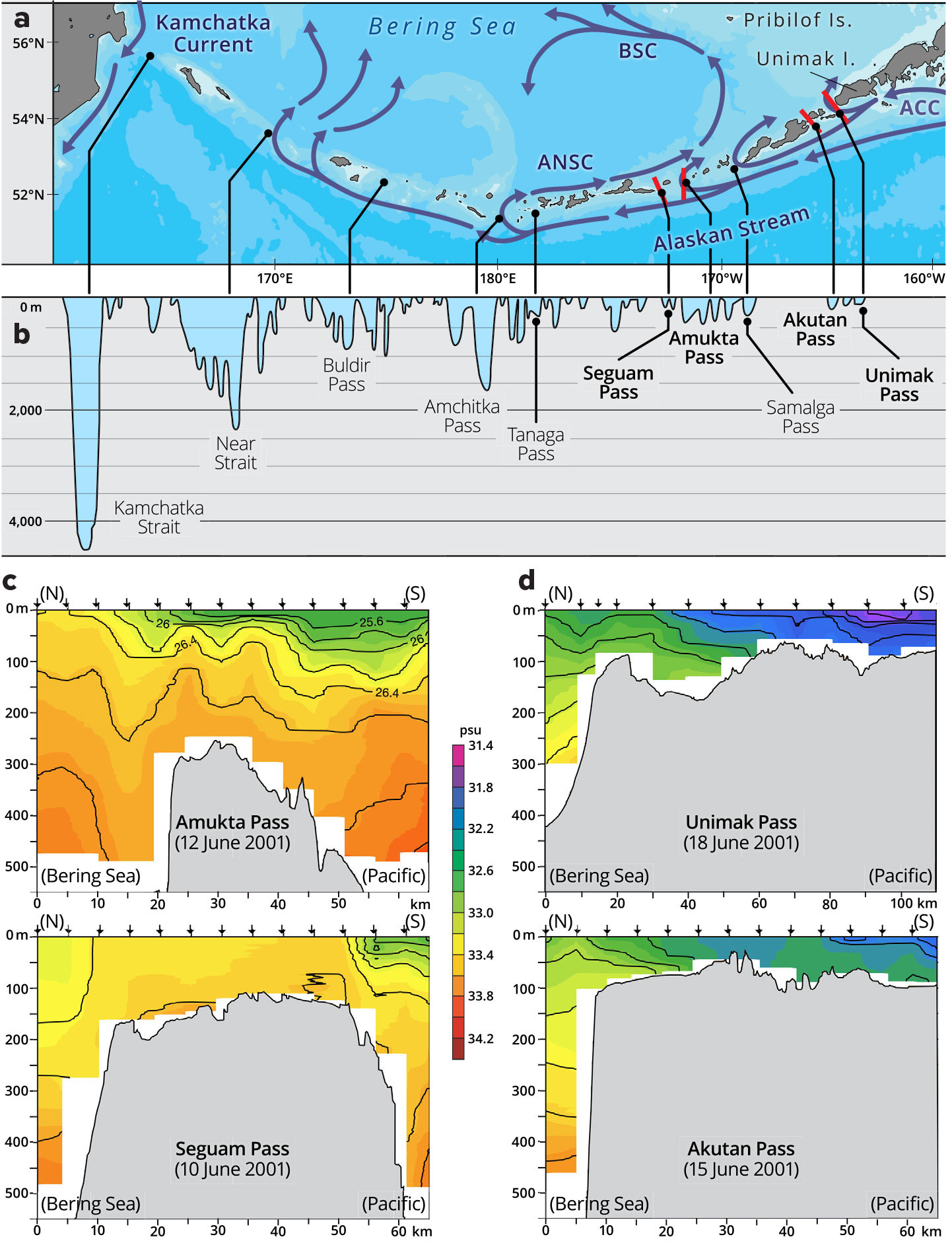
FIGURE 4. Surface currents (a) and bathymetry (b) along the Aleutian archipelago. Salinity sections show the extent of mixing over sills in deep (c) versus shallow (d) passes. Red bars in (a) show the locations of the salinity sections, and arrows over each section indicate the locations of CTD casts. Adapted from Ladd et al. (2005) and Stabeno et al. (2005b). > High res figure
|
Aleutian Passes
The dramatic decline of western Steller sea lions (Eumetopias jubatus) in 1970–1990 resulted in 2001 congressional legislation that called for investigation of possible causes. In 2001 and 2002, EcoFOCI collaborated with other NOAA and academic partners on the first comprehensive multidisciplinary study of the eastern and central Aleutian Passes, with joint funding by NOAA and NSF (Stabeno et al., 2005a). EcoFOCI focused on physics, chemistry, and lower trophic production to provide information that was linked to studies conducted by outside investigators on corals, fish, birds, and marine mammals inhabiting the archipelago.
The Aleutian Passes allow water exchange between the Pacific Ocean and the Bering Sea. The depth and width of the passes generally increase from east to west (Figure 4), with Kamchatka Strait in the west being the only pass that permits exchange of deep (>2,000 m) water. Net transport is northward, with strong northward flow on the east side of each pass and weaker, or nonexistent, southward flow on the west sides (Cokelet et al., 1996; Stabeno et al., 2005b). Tidally induced vertical mixing is evident over the sills in the passes, to depths greater than 200 m from fall to spring (Stabeno et al., 2005b), thereby introducing salt and nutrients to surface waters (Figure 4; Mordy et al., 2005) and promoting primary production downstream in the northeastward flowing Aleutian North Slope Current (first identified in Reed and Stabeno, 1999).
A major finding of the Aleutian Passes study was partitioning of the Aleutian ecosystem at Samalga Pass (Ladd et al., 2005; Stabeno et al., 2005b), as reported in a special issue of Fisheries Oceanography and summarized by Stabeno et al. (2005a) as follows. East of Samalga, the GOA shelf widens, is fed by the ACC, and has a shelf-like ecosystem that includes more abundant neritic zooplankton species that serve as prey for short-tailed shearwaters; abundant fish species with euphausiids serving as an important prey species; pollock as the main prey item for sea lions; and an absence of sperm whales. To the west, the shelf is narrow, the passes are fed by the Alaskan Stream, and the ecosystem is more oceanic, including oceanic zooplankton as prey for northern fulmars and auklets, fewer fish species with copepods and myctophids as primary prey, Atka mackerel as the primary prey for sea lions, and a diversity of cold-water corals and sponges.
Climate and Recruitment
The 1980s and 1990s represented a period of major progress in our understanding of climate variability of the North Pacific. The PDO was identified, along with its correspondence to biological variables (Mantua et al., 1997). Under the auspices of EcoFOCI, Stabeno et al. (2004) followed up those earlier studies with a review of the mesoscale oceanography of the northern GOA vis-à-vis the larger-scale atmosphere-ocean system. But the North Pacific atmosphere-ocean system, and associated ecosystem responses, are arguably not as simple as first described; examples of these complications were found under the broad umbrella of EcoFOCI and related programs.
One finding was that the PDO was often, but not always, the leading source of variability in the North Pacific (Bond et al., 2003). Di Lorenzo et al. (2013) summarized linkages between various elements of the ecosystem and the second-leading mode of North Pacific Ocean variability, the North Pacific Gyre Oscillation. Additional advances were made in appreciating the importance of advection in the Pacific Subarctic Gyre (Hristova et al., 2019). Recent research shows that North Pacific climate patterns, such as the PDO, have different expressions in different multi-year periods. This is evident in the spatial distributions of the various patterns of sea surface temperature and sea level pressure anomalies, and in their relationships to fishery catches and other ecosystem indicators (e.g., Litzow et al., 2020).
The role of subsurface temperatures represents one of EcoFOCI’s recent contributions to the fisheries-oceanography understanding of the GOA. A marine heatwave, called “the Blob,” produced anomalously warm waters off the US West Coast and in the GOA in 2014–2016 that led to closure of a major federal fishery—Pacific cod (Gadus macrocephalus) in 2020.
As the nation’s marine ecosystem stewards, NOAA has conducted trawl-net surveys of groundfish (fish that live on or near the bottom) in the GOA since 1990. Measurements from those surveys show that in 2015, the average bottom temperatures (<500 m deep) in the western (central) gulf regions were 1.25°C (0.88°C) warmer than average. EcoFOCI and other regional researchers studied six commercial groundfish species in different life stages to determine whether individuals moved to more favorable habitats during warming (Yang et al., 2019), while recognizing that fishes are also affected by predation from larger fishes, prey availability, and metabolic demands.
Most species showed complex responses to warming that were related to species-specific ontogeny and partitioning within temperature-depth habitats. In the central GOA, all sizes of Pacific cod shifted to deeper, colder water during the heatwave. Nevertheless, they were still in a habitat that was warmer than one they had, on average, occupied over the previous decade. Pacific cod biomass declined substantially due to an increase in metabolic demand, a reduction in prey supply during the heatwave, and continued fishing pressure. Historically low recruitment concurrent with the heatwave resulted in the 2020 closure of the federal Pacific cod fishery in the GOA, the second largest commercial groundfish fishery in Alaska (Barbeaux et al., 2020).
Future Directions
The GOA marine heatwave of 2014–2016 and the subsequent extreme biological responses across the ecosystem (e.g., fish stock collapses, seabird mass mortalities, increase in harmful algal blooms, marine mammal mortality events) have drawn intense focus on the future of the GOA region and connections among climate, oceanography, and ecosystems. Societal interests are being impacted, and accelerating anthropogenic climate change is certain to continue driving complex and potentially unprecedented ecosystem variability in the near future.
Sustained observations, integration among research disciplines, and new observing techniques are essential to improving our understanding of future climate-biology relationships and assessing vulnerability of stakeholders to shifting ecosystems in the GOA and Aleutian region. EcoFOCI is set up to meet those challenges.
The EcoFOCI partnership was in many respects ahead of its time. Prior to EcoFOCI, PMEL and AFSC conducted independent but overlapping research. Their partnership brought a whole-ecosystem context to fishery science—and a fishery context to oceanographic science. This partnership was formed a decade or more before ecosystem-based fisheries management became the aspirational standard in the United States and the world. Now, after nearly 40 years of EcoFOCI collaboration, Alaskan fisheries are among the best managed and most understood, thanks in part to constructive relationships among fishing industry, management, and marine scientists across disciplines, including EcoFOCI (Figure 5). In the future, we expect these partnerships to become increasingly important as high-latitude marine ecosystems are undergoing rapid, unprecedented change. Maintaining the blue economy while building coastal and climate resilience requires continued monitoring, incorporating new technologies, and updating models to keep pace with these evolving ecosystems in this highly dynamic region.
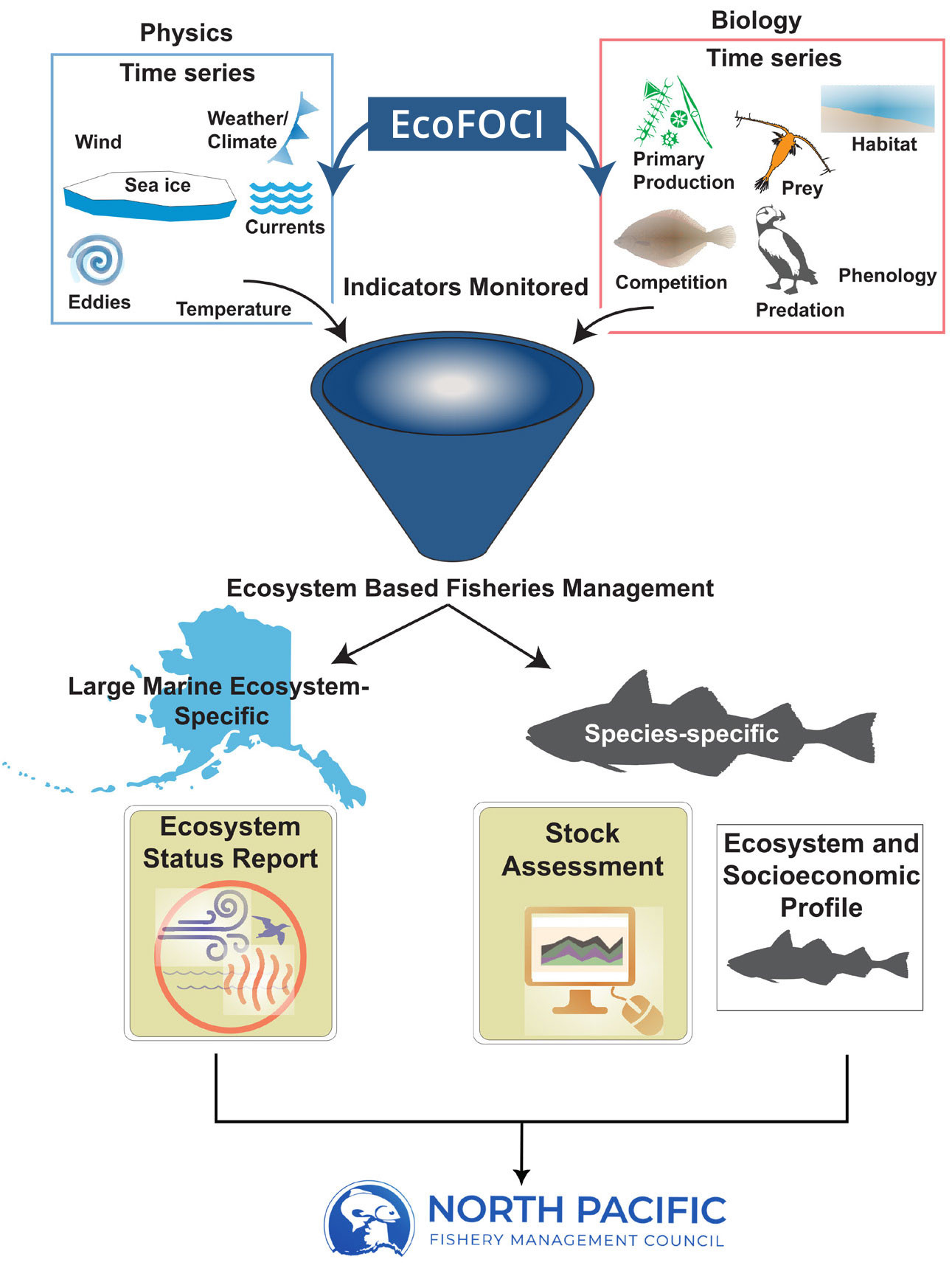
FIGURE 5. Schematic representation of information flow from EcoFOCI to ecosystem scientists, stock assessment scientists, and the North Pacific Fishery Management Council (NPFMC). Life history information, experimental data, and data collected at sea and from satellites are used along with models to understand the operative mechanisms controlling fish recruitment. Predictions of future recruitment rely on indicators produced from the long time series data (physics outlined in blue, and biology in red). Indicators are monitored and updated annually. These indicators contribute to ecosystem-based fisheries management at the large marine ecosystem level via the NOAA Fisheries Marine Ecosystem Status Reports and at the fishery stock level via the Stock Assessment (either as part of the required risk table or in the operational model) and in the Ecosystem and Socioeconomic Profile. These products are then provided to the NPFMC. > High res figure
|
ACKNOWLEDGMENTS
This paper is dedicated to the memory of Robert L. Charnell and Patrick Laird. These PMEL oceanographic researchers were lost at sea with M/V Holoholo in December of 1978 in the Alenuihaha Channel off the island of Hawai‘i. Profound thanks to the scientists, technicians, ship personnel, academic and NOAA partners that have contributed to EcoFOCI over the past four decades. Special thanks to the EcoFOCI leadership teams through the years including those mentioned in the Origins of EcoFOCI section as well as Jeff Napp and Janet Duffy-Anderson at AFSC and Phyllis Stabeno at PMEL. Thanks to S. Battle and Ali Deary for the beautiful figures, and for reviews by C. Ladd, Jeff Napp, and one anonymous reviewer. This research is supported by NOAA Research and Fisheries and partially funded by the Cooperative Institute for Climate, Ocean, and Ecosystem Studies (CICOES) under NOAA Cooperative Agreement NA20OAR4320271. This is contribution EcoFOCI-1035 to EcoFOCI, 2023-1253 to CICOES, and 5479 to PMEL. The scientific results and conclusions, as well as any views or opinions expressed herein, are those of the authors and do not necessarily reflect those of OAR or the Department of Commerce.