Introduction
The Pacific Arctic region (PAR) is important both regionally and globally. It supports the region’s climate functions, ecosystems, and social systems, all of which are inexorably linked to the Indigenous communities and the physical, chemical, and biological functioning of the Bering, Chukchi, and Beaufort Seas. These systems derive their regional structures and characters as direct consequences of ocean currents (Woodgate and Peralta-Ferriz, 2021) that deliver heat, nutrients, and carbon northward across the PAR (Figure 1). Within the region, the Alaskan Arctic marine environment has undergone widespread warming in recent decades (Danielson et al., 2020), and the area’s decreasing sea ice is associated with ecological responses and adaptations by Arctic coastal residents (Grebmeier et al., 2006; Huntington et al., 2020; Hauser et al., 2021). Monitoring this entire area for all responses is beyond the scope of a single observational effort, yet much may be achieved by combining information gathered by different programs, especially long-term in situ studies. Together, such efforts yield a pan-region interdisciplinary synergy. In this article, we aim to demonstrate the breadth of existing monitoring efforts in the US Arctic, which extends from the eastern Bering Sea shelf to the Beaufort Sea shelf. The long-term observation programs we introduce provide time-series data that are critical for quantifying ecosystem changes, anticipating future conditions, and understanding linkages between loss of sea ice, alterations in oceanographic and biogeochemical conditions, and effects on people and all trophic levels from microbes to apex predators.
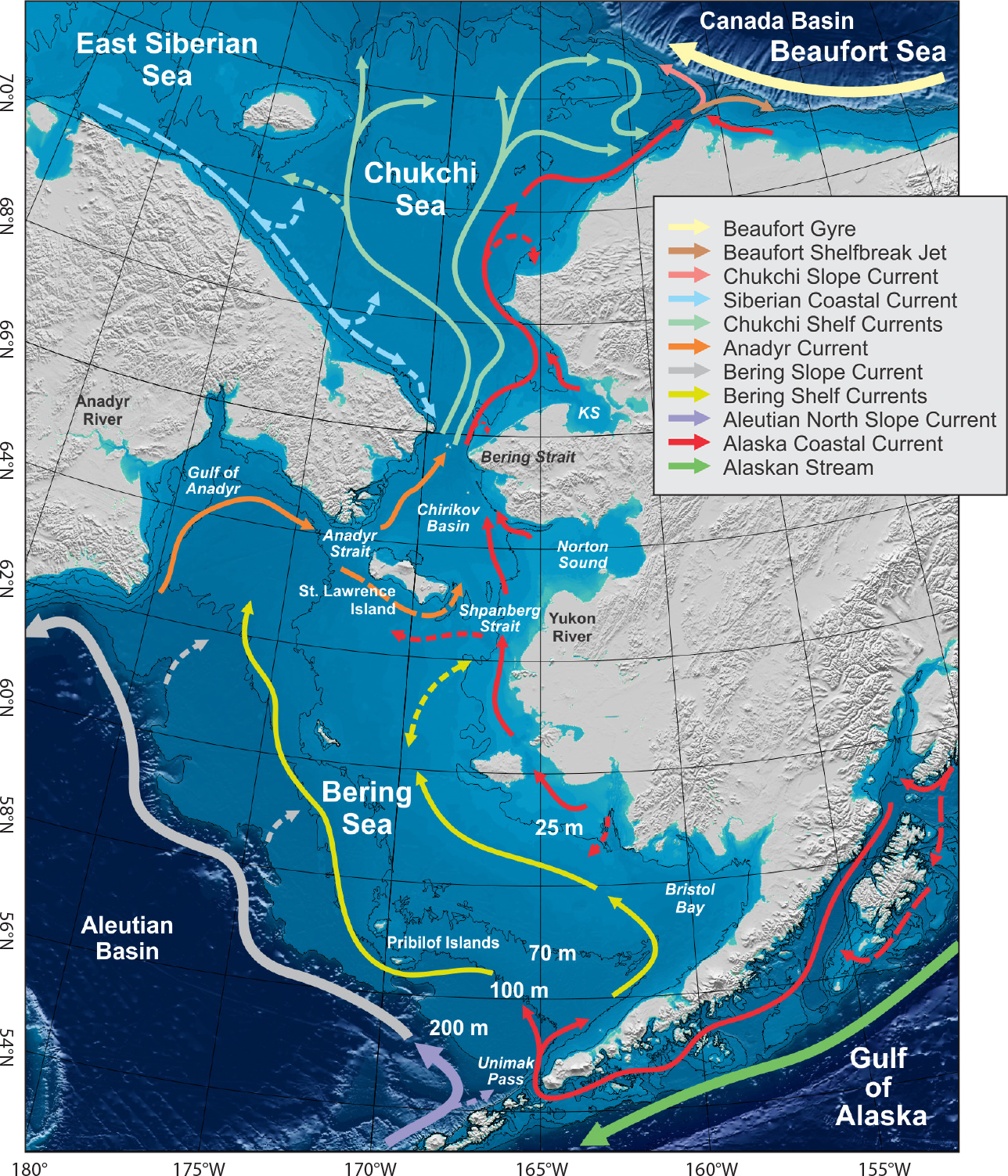
FIGURE 1. Map of the region typically called the Pacific Arctic, showing place names and an idealized depiction of the mean oceanic currents. KS = Kotzebue Sound. > High res figure
|
One of the pressing needs for evaluating climate change impacts on the marine ecosystem in the Arctic (and globally) is the need for sustained observations of change. Biological observations cannot be automated to the same extent as many physical measurements and as a result, there is far less scientific documentation of how biological systems are changing and/or adapting due to environmental changes. Sustained investment of public funds that supports ongoing monitoring efforts, along with the use of these data in resource management decisions, provides an indication of their societal importance.
Here, we describe a selected set of 17 independently funded, organized, and operated long-term monitoring programs (Table 1) that, while formally uncoordinated as a whole, in practice represent a rich, ongoing, and frequently updated suite of data collections that together characterize many aspects of the Alaskan Arctic marine systems. We include only long-term, in situ observational monitoring efforts. Together, they document aspects of ocean and ice physics, nutrient and carbonate chemistry, and all trophic levels. Some efforts make observations only in the open water season while others continue year-round. Our selection represents a diversity of funding agencies, geographic coverage, and observing techniques. These efforts document environmental and ecosystem structure and change across spatial scales as large as the expanse of the Bering-Chukchi-Beaufort shelves and timescales spanning hours to decades. They include vessel-based surveys, autonomous platforms, and coastal community-based efforts. The latter provide a unique, invaluable, and much longer-term perspective on system change based on deep insight into the structure and functioning of the Arctic system by drawing on long-developed local traditional Indigenous knowledge (Eicken et al., 2021).
TABLE 1. Selected in situ long-term observing systems active in the Alaskan Arctic in recent years, along with program type, year of first sampling, observational focus, and funding sources. Most programs listed here have gaps of one year or more since their beginning. > High res table
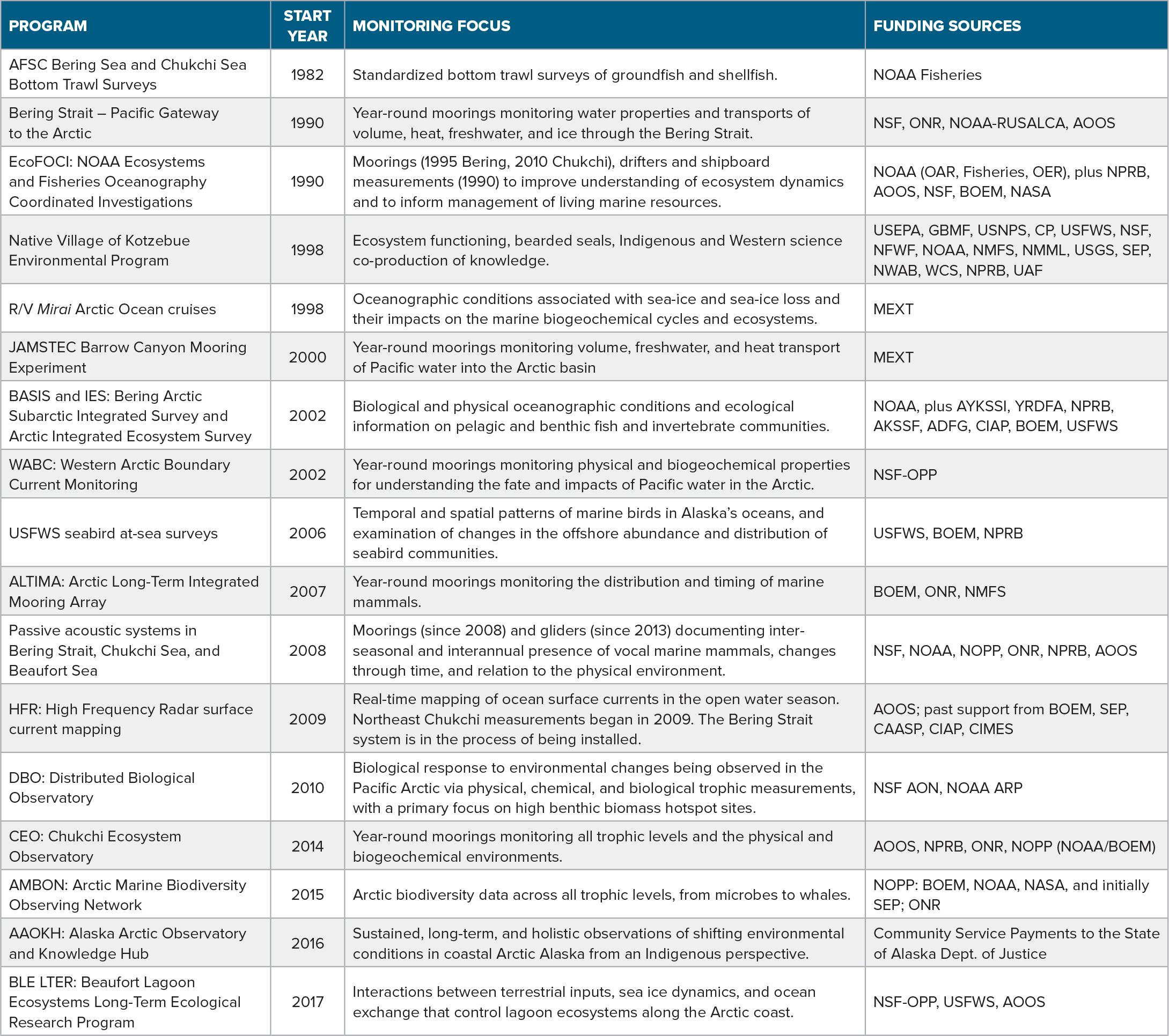
|
TABLE 2. Representative observing system successes and insights from the programs listed in Table 1. > High res table
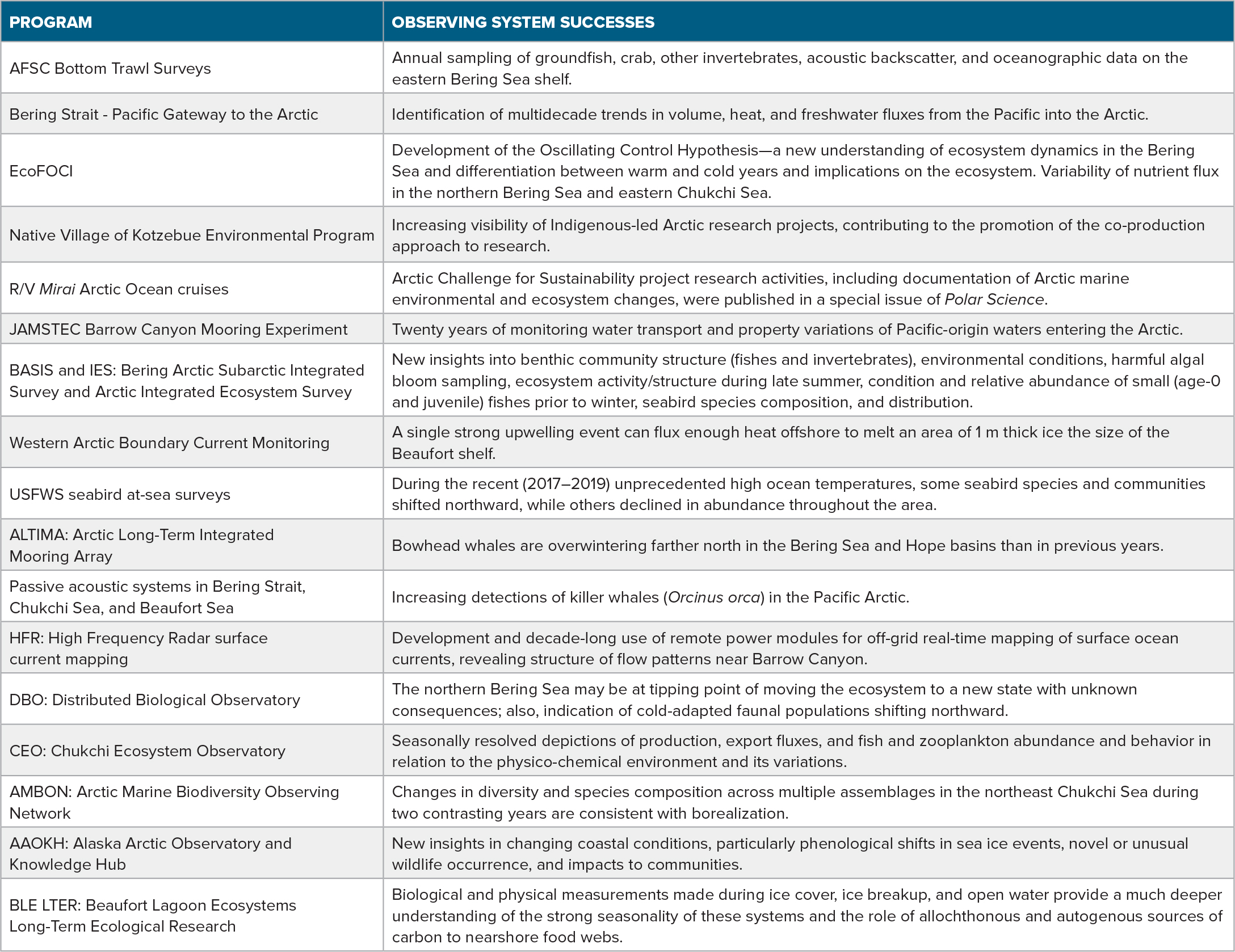
|
This discussion does not include off-shelf studies and individual process studies (typically one to three years of fieldwork) and larger process-oriented research programs (typically three to ten years of fieldwork). Nevertheless, process studies play a key role in advancing our understanding of the ecosystem, provide baseline data that underlie ongoing monitoring, and inform monitoring priorities and approaches. In turn, the monitoring programs help define questions that require the attention of new process studies. Prominent ecosystem-focused PAR process study programs conducted in recent decades include, but are not limited to, the following efforts (acronyms and field years are given in parentheses): Processes and Resources of the Bering Sea Shelf (PROBES; 1976–1982), Inner Shelf Transfer and Recycling (ISHTAR; 1983–1989), Western Arctic Shelf-Basin Interactions (SBI; 2002–2006), Bering Ecosystem Study (BEST; 2007–2011), Bering Sea Integrated Ecosystem Research Program (BSIERP; 2007–2011), Russian-America Long-term Census of the Arctic (RUSALCA; 2004–2012), Chukchi Sea Environmental Studies Program (CSESP; 2008–2015), and Arctic Integrated Ecosystem Research Program (Arctic IERP; 2017–2019).
Although independently executed, each of the monitoring programs described here benefits from inter-program coordination that includes community outreach, planning, leveraged field logistics, data sharing, and collaborative data analyses. Avoiding marine domain conflict with subsistence hunters is a top priority for Alaska-region scientific field efforts, and clear and open communication with subsistence harvest co-management groups and village tribal councils is critical for maintaining productive and respectful relationships. Scientific monitoring benefits from local co-production of knowledge partnerships carried out with Indigenous coastal communities (Hauser et al., 2021). Co-management group meetings, such as those of the Alaska Eskimo Whaling Commission, help researchers communicate with community representatives. Research coordination is also fostered by the Alaska Marine Science Symposium (AMSS), a conference held annually in Anchorage that attracts ~1,000 marine researchers and provides a forum for communication across the Pacific subarctic and Arctic research communities. In addition to other national and international scientific meetings, meetings of the Pacific Arctic Group (PAG), the North Pacific Marine Science Organization (PICES), and the Ecosystem Studies of the Subarctic and Arctic Seas (ESSAS) help coordinate PAR field research and scientific collaborations on an international basis.
While many monitoring programs (Table 1) have different objectives and geographical foci, we find commonality in approaches, motivations, and innovations. One means of assessing changes in the marine environment is to examine the distribution of flora and fauna that are directly influenced by environmental changes. In the Arctic, marine mammals and seabirds have been proposed as ecosystem sentinels of change (Moore and Kuletz, 2019), and passive acoustic sampling has proven to be a robust means of detecting species-specific presence of vocalizing marine mammals throughout the year (Stafford et al., 2021). Similar approaches are applied to planktonic, pelagic, and benthic communities resolved by time-series sediment traps, water samplers, photographic and active acoustic imaging systems, nets, and benthic grabs, cores, and trawls (see citations later in the text). Specific results and additional references for the programs discussed below and listed in Table 1 are included in the online supplementary materials.
Community-Based and Coastally Focused Programs
Coordinated community-based observing efforts, such as the Alaska Arctic Observatory & Knowledge Hub (AAOKH), provide distributed, sustained, long-term, and holistic observations of shifting environmental conditions in coastal Arctic Alaska from an Indigenous perspective (Figure 2a). Iñupiaq people in northern Alaska have been monitoring coastal changes for millennia and are among the first to experience and detect changes in the environment, given their deep connections to place and integral reliance on traditional marine and coastal resources. AAOKH is an observing network whose primary goal is to provide communities with the tools, resources, and scientific support to share their expertise and Indigenous knowledge through observations of changing coastal conditions and associated impacts on their access to traditional marine resources. Iñupiaq local experts, termed “observers,” regularly document environmental conditions and community events, including weather; sea ice or ocean conditions and travel safety; coastal erosion; river freeze-up and breakup; and marine or coastal fish, bird, and wildlife sightings and harvests. Some observers also seasonally measure aspects of coastal physical (e.g., temperature, salinity) and biological (e.g., chlorophyll a fluorescence) oceanographic or landfast sea ice conditions (e.g., ice mass balance, ice edge location, sea ice thickness).
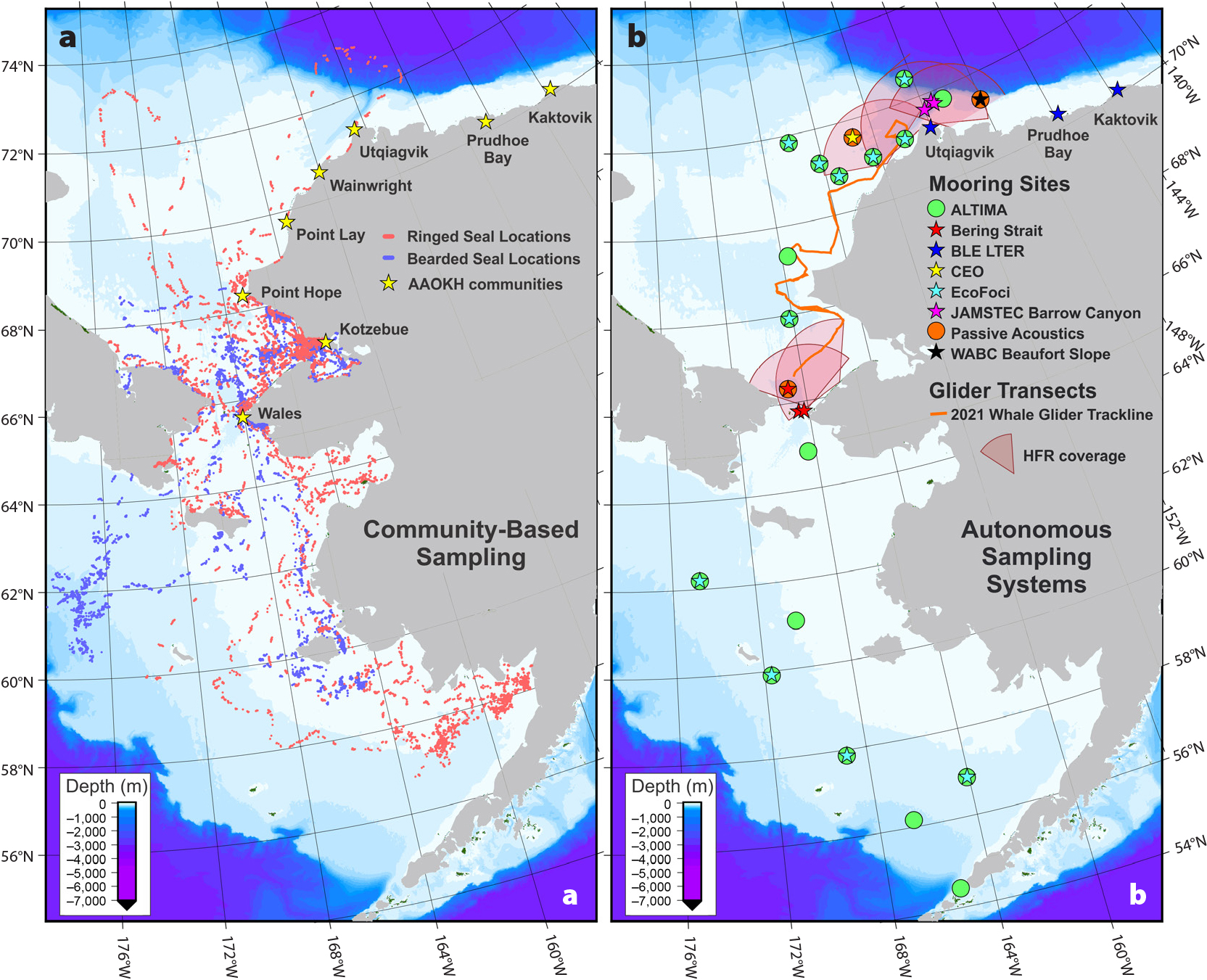
FIGURE 2. (a) Locations of current or past Alaska Arctic Observatory & Knowledge Hub (AAOKH) communities (yellow stars) and GPS locations of ringed (red) and bearded (blue) seals between September 2009 and July 2010. Seals were captured and tagged near Kotzebue during fall 2009 as part of a cooperative project between the Native Village of Kotzebue Environmental Program and the Alaska Department of Fish and Game. (b) Autonomous measurements: mooring sites (symbols), high frequency radar coverage (shaded arcs), and a 2021 passive acoustic glider track (orange line). Circles denote passive acoustic recorders, and stars denote multidisciplinary oceanographic moorings. Not shown: Saildrone tracklines that criss-cross the US Bering/Chukchi shelves. > High res figure
|
Coordinated and year-round observations from AAOKH observers collectively provide a broad-scale and synoptic view of changing coastal sea ice and ocean conditions, and ultimately impacts, at the coastal community scale (Eicken et al., 2021). Recent themes emerging from AAOKH observations document abrupt changes in landfast sea ice that affect travel safety, shoreline erosion affecting infrastructure, warming ocean and air temperatures, and shifts in wind and weather patterns. AAOKH observers also identify anomalous conditions, such as novel species or shifts in the seasonal occurrence of regular species. Environmental changes are often linked to food security and impacts on traditional lifestyles. The AAOKH network evolved out of a predecessor program called the Seasonal Ice Zone Observing Network (SIZONet), with observations dating as early as 2006 and encompassing several western and northern Alaska communities (Eicken et al., 2014). The shared and ongoing AAOKH-SIZONet database currently includes >8,000 observations that encompass local and Indigenous perspectives of coastal change (Adams et al., 2013).
The Native Village of Kotzebue Environmental Program (NVK EP) facilitates and undertakes research on the ecology of Kotzebue Sound through a combination of Indigenous knowledge and skill sets with western science and scientists using co-production of knowledge approaches (Whiting et al., 2011; Hauser et al., 2021; Mahoney et al., 2021). Research activities have targeted the study of fish and other wildlife species found in Kotzebue Sound, including their health, behavior, and impacts resulting from the rapidly changing climate. NVK EP efforts also focus on ice seals, beluga whales, and sheefish (inconnu); the coastal lagoon system and its relationship to the ecology of the marine ecosystem, with a particular focus on whitefish species; contaminants and nutrients found in species of high subsistence value (e.g., ice seals, sheefish, whitefish); the physical environment of the sound, including sea ice, snow pack, water quality (physical, chemical, and biological), and currents (circulation and hydrographic structure); emergent harmful algal blooms; and the contribution of the Sound to the annual subsistence harvest of the people of Kotzebue (Qikiqtaġruŋmiut).
The Beaufort Lagoon Ecosystems Long Term Ecological Research (BLE-LTER) program is focused on interactions between terrestrial inputs, sea ice dynamics, and ocean exchange that control lagoon ecosystems along the Arctic coast over seasonal to multidecadal timeframes (e.g., Bonsell and Dunton, 2018). This program, which incorporates Indigenous community participation and support, is broadly relevant to understanding ecosystem dynamics at the Arctic’s land-sea interface. The BLE-LTER fills a need for strongly interdisciplinary and ecology-focused studies in the shallow coastal waters of the Arctic. Overarching goals are to improve our fundamental understanding of land-sea coupling and to project how climate change is altering coastal ecosystems.
BLE-LTER core program measurements include seasonal data collections for physical, biogeochemical, and biological properties in coastal lagoons across 500 km of coastline at three nodes distributed along the Alaskan Beaufort Sea coast at Utqiaġvik, Prudhoe Bay, and Kaktovik (Figure 2b). Two lagoons within each node are sampled during the periods of ice cover (April), sea ice breakup (June), and open water (August). In addition, the program collects continuous, high frequency measurements at multiple mooring sites at all three nodes. BLE-LTER inventory, process, and mooring observations are critical to understanding ecosystem responses to changes in sea ice extent and duration, coastal erosion, and freshwater inputs.
Vessel-Based Monitoring
Research programs from multiple Pacific Rim countries annually conduct research cruises across the Bering-Chukchi shelves and into the Arctic Basin. With some exceptions, research cruises north of Bering Strait are limited by sea ice to the months of July through October.
The Distributed Biological Observatory (DBO) is a change detection network that is evaluating alterations in the PAR in the context of declining seasonal sea ice, warming water temperatures, stratification changes, and other processes that are impacting all aspects of the overall ecosystem (Moore and Grebmeier, 2018). Initiated in 2010, the overarching goal of the DBO collaboration network is a comprehensive implementation of standardized ocean sampling as research cruises from the international community transit through the PAR. The surveys focus on regions of high productivity and biodiversity (five regions initially, later expanded to eight) that extend from the Northern Bering Sea to the Chukchi and Beaufort Seas (Figure 3a). Sampling includes seawater temperature, salinity, currents, nutrients, chlorophyll, carbon products, zooplankton, benthic fauna and sediments, and observations of seabirds (Kuletz et al., 2019) and marine mammals. The DBO is an international partnership involving Canada, China, Japan, Korea, Russia, and the United States, and many agencies within those countries, with collaborations for satellite observations, moorings, and autonomous sensor sampling in the DBO regions. Sampling is focused on transects centered on locations of high productivity, benthic biomass, and rates of biological change, using multiple international vessels annually. This DBO sampling concept is presently being expanded to other portions of the Arctic, including the Canadian Beaufort Sea, Baffin Bay, Davis Strait, waters near Svalbard, and possibly the Laptev Sea through German-Russian cooperative programs.
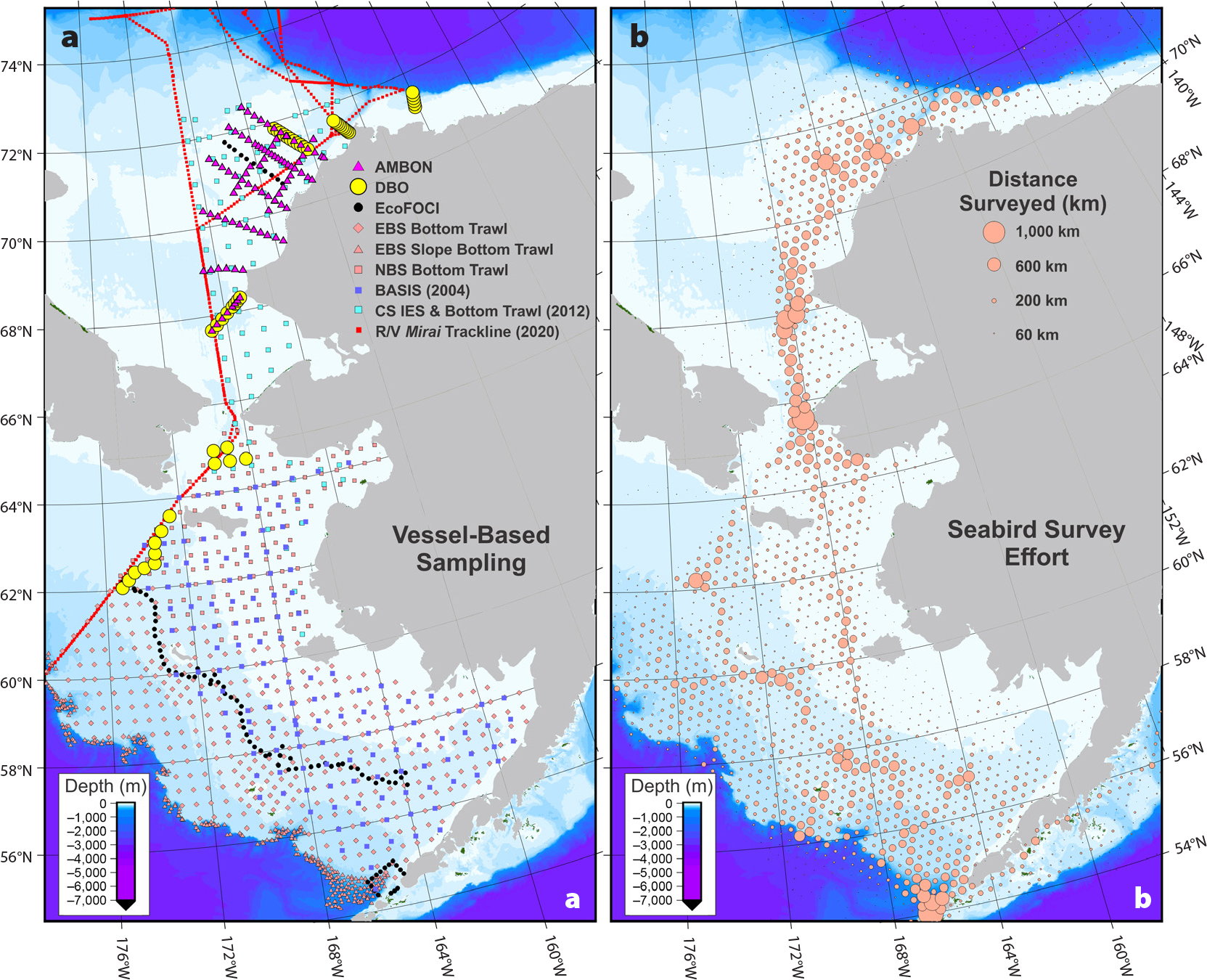
FIGURE 3. (a) Vessel-based survey stations (symbols) and the 2020 R/V Mirai trackline as a representative effort in one recent year (red squares). (b) Vessel-based seabird survey effort (30 km grid cells) from 2006 to 2020 showing total kilometers of survey transect per grid cell. > High res figure
|
In the early 2000s, the Japan Agency for Marine-Earth Science and Technology (JAMSTEC) initiated studies to understand the oceanic halocline structures that help maintain sea ice distribution in the PAR. With decreasing sea ice in recent years, the research foci shifted to studying the physical oceanographic conditions associated with sea ice loss and associated impacts on marine biogeochemical cycles and ecosystems (e.g., Nishino et al., 2016). Resulting Arctic-focused projects since 2011 include the Arctic Climate Change Research Project under the Green Network of Excellence (GRENE; 2011–2016), Arctic Challenge for Sustainability project (ArCS; 2015–2019), and Arctic Challenge for Sustainability II project (ArCS II; 2020–2025). Although the GRENE project was mainly carried out by natural scientists, ArCS and ArCS II are more interdisciplinary, reflecting issues that also concern social scientists, decision-makers, practitioners, and the general public, particularly Indigenous residents of the Arctic coastal communities.
Observations of biological, chemical, and physical oceanographic conditions and ecological information on pelagic and benthic fish and invertebrate communities are provided by the NOAA Alaska Fisheries Science Center (AFSC), Bering Arctic-Subarctic Integrated Survey (BASIS; see station grid in Figure 3a). These surveys assist efforts to understand how the Bering Sea ecosystem is responding to loss of seasonal sea ice (Farley et al., 2020). Late-summer surveys occur in the southeastern Bering Sea biennially (even years) in late summer, and annually in the northern Bering Sea. In the Chukchi Sea, the Arctic Integrated Ecosystem Survey (IES) occurs less frequently (2003, 2007, 2012, 2013, 2017, 2019). Sampling at each station includes CTD profiles and discrete water samples for chlorophyll, nutrients, and environmental DNA (eDNA), net tows for zooplankton, and trawls for pelagic fishes, including young-of-the-year gadids, forage fishes, and juvenile salmonids. Benthic community ecology sampling in the Chukchi Sea began in 2017 and on BASIS surveys during 2018, targeting young-of-the-year fishes, crabs, clams and other invertebrates, and sediments.
The Groundfish Assessment (GAP) and Shellfish Assessment (SAP) Programs in the Resource Assessment and Conservation Engineering (RACE) division of the NOAA Alaska Fisheries Science Center (AFSC) have been conducting standardized bottom trawl surveys of groundfish and shellfish in the Bering Sea since 1982. The main goal of these bottom trawl surveys is to assess and monitor the conditions of these populations in the Bering Sea. The AFSC conducts four separate regional bottom trawl surveys: Eastern Bering Sea shelf (EBS), EBS slope (EBSS), Northern Bering Sea (NBS), and Chukchi Sea (CS) surveys. Data collected are critical for managing fisheries, ecosystem monitoring, and advancing research on groundfish and crab populations throughout the region. Abundance, age and size composition data, and other biological and environmental oceanographic information from the bottom trawl surveys are used by AFSC, the North Pacific Fishery Management Council, and the Alaska Department of Fish and Game to manage groundfish and crab stocks. These data also build the basis for ecosystem models to improve scientific understanding of the Bering Sea ecosystem and to forecast potential changes to the ecosystem in response to the changing climate (Stevenson and Lauth, 2019).
In addition to population-level data, the AFSC bottom trawl surveys produce catch-per-unit-effort data on fish and invertebrate density that provide information on the distribution of marine fauna. These data have been critical in detecting and monitoring distribution shifts of groundfish in the region (Stevenson and Lauth, 2019). Survey efforts also include studies of genetics, diet, fish condition, acoustic backscatter, ecosystem indicators, and fish tagging. Oceanographic data routinely collected on the bottom trawl surveys include temperature, salinity, depth, and irradiance.
Seabirds are another upper trophic level indicator of marine ecosystem conditions. US Fish and Wildlife Service (USFWS) at-sea surveys (Figure 3b) describe temporal and spatial patterns and trends of marine birds in the PAR and examine changes in offshore seabird communities. The data are submitted to the North Pacific Pelagic Seabird Database for a variety of conservation, science, and management applications, and to inform environmental assessments of marine planning areas. They provide an upper trophic level component to multidisciplinary research and monitoring projects. Ultimately, the goals are to identify factors causing observed changes and to facilitate adaptive management for seabird populations. Ancillary observations of marine mammals are also recorded but are not used to estimate species’ densities or abundance.
The seabird surveys depend on collaborations with vessel-based research programs (Table 1). By doing so, the USFWS has been able to leverage interagency and external grants to obtain greater survey coverage. Although project-specific data can provide valuable insights, combined data from multiple programs and years, often using repeated sampling stations (e.g., the DBO array; Kuletz et al., 2019), allow researchers to more fully examine seasonal and long-term patterns and changes in the seabird community. For example, Kuletz et al. (2020) found a northward shift in some seabird species and communities and declines in others during the exceptionally warm 2017–2019 period.
Complementary to the population assessment efforts, the Arctic Marine Biodiversity Observing Network (AMBON) contributes Arctic biodiversity data to the growing knowledge of Arctic systems across all trophic levels from microbes to whales (Iken et al., 2019). High biodiversity is thought to foster stable ecosystems because of the higher number, complexity, and redundancy of ecosystem functions that a larger number of coexisting species can support. Hence, contributing an Arctic perspective to national and international networks of Marine Biodiversity Observation (MBON) programs is a significant step forward in understanding global ocean processes and in managing, regulating, and mitigating human-ocean interactions. Vessel-based sampling occurs over several cross- and along-shelf environmental gradients from the southern to the northeastern Chukchi Sea or is conducted opportunistically in conjunction with other research efforts. Collections include microbes or eDNA from surface and bottom waters, small and large zooplankton from net collections, benthic macrofauna from grab samples, epibenthic invertebrates and demersal fishes from small beam trawls, pelagic fishes from midwater trawls, and shipboard observations of seabirds and marine mammals. Concurrently, water column and sediment environmental parameters are collected for relation to patterns in biodiversity. A goal of the AMBON project is to work collaboratively with other research efforts to optimize the production of new knowledge. For example, the AMBON project builds on prior studies in the Chukchi Sea and collaborates closely with the Chukchi Ecosystem Observatory (CEO) program, with the USFWS seabird at-sea surveys, and the DBO program.
Autonomous Platform-Based Monitoring
Autonomous sampling platforms (Figure 2b) can provide cost-effective means to monitor the marine realm using fixed installations and mobile autonomous uncrewed vehicles (AUVs, e.g., gliders and Saildrones). Moorings provide high temporal resolution data throughout the year, including the under-sampled fall, winter, and spring seasons when sea ice inhibits ship-based sampling and darkness precludes bird and mammal visual observations. Land-based high frequency radar (HFR) systems produce hourly maps of surface ocean currents that are used in oil spill responses, missing boater searches, and numerous scientific applications. While sensors that reliably measure the physical environment have been available for decades, recent advances in instrument technology now allow us to autonomously sample the marine ecosystem across many disciplines and including all trophic levels.
Since 1990, moorings in the Bering Strait (maintained by the University of Washington) have measured the key physical oceanic parameters of Pacific waters flowing towards the Arctic, quantifying velocity, temperature, salinity, and fluxes of volume, heat, and freshwater. Flow properties vary greatly both seasonally and interannually, driving the need for year-round measurements. Since 2002, measurements have also been made of ice velocity and thickness, and since 2007, there have been separate measurements of upper layer temperature and salinity. The Diomede Islands split Bering Strait into two channels—one in the United States and one in Russia. Originally, moorings were placed in both channels and at a site 35 km north of the strait proper, a location found to give a useful average of the flow properties through both channels. The mooring placements (Figure 2b) allow quantification of the flow even when access to the Russian channel is not possible. The full time series shows increasing northward flow (~70% increase since 2001), warming (~0.1°C yr–1 in summer), longer open water duration (~1.5 days per year), and dramatic freshening, especially in winter (~0.03 psu yr–1; Woodgate, 2018; Woodgate and Peralta-Ferriz, 2021). These data represent one example of long-term monitoring that revealed signals not anticipated at the program onset; documentation of these changes is now foundational to our understanding of PAR changes across physical, chemical, and biological systems.
JAMSTEC has maintained subsurface oceanographic moorings at the mouth of Barrow Canyon in the Northeast Chukchi Sea since 2000, monitoring the volume, freshwater, and heat transport of Pacific-origin water into the Arctic Basin. Barrow Canyon, a major conduit for this flow, is also where deeper Atlantic-origin water sometimes upwells onto the Chukchi shelf. The moorings assess winter water fluxes, which strongly influence the Arctic Basin environment by ventilating the interior halocline and providing nutrients that spur primary production, while summer water is a predominant source of heat and freshwater (e.g., Itoh et al., 2013). Annual mean volume transport through Barrow Canyon (0.45 Sv) represents 55% of the long-term mean Pacific Water inflow through Bering Strait (Itoh et al., 2013) and ~94% of the Bering Strait transport during summer. Over the last decade, warm Pacific summer water has significantly contributed to both sea ice melt in summer and a decrease in sea ice formation during winter.
As part of the US National Science Foundation-supported Arctic Observing Network, the Woods Hole Oceanographic Institution has maintained a year-round mooring on the Beaufort continental slope in the western Arctic boundary current (WABC), along with biennial autumn ocean current shipboard surveys. The WABC, a critical conduit of Pacific water, advects elevated concentrations of nutrients, chlorophyll, dissolved and particulate organic carbon, and zooplankton towards the Canadian Arctic Archipelago (Pickart et al., 2013). Bowhead and beluga whales congregate near the current along the outer shelf and continental slope of the Beaufort Sea (Stafford et al. 2021). Due to wind-driven upwelling/downwelling and hydrodynamic instability, the WABC fluxes a substantial fraction of its mass, heat, freshwater, and biogenic content offshore, impacting the Arctic interior water column, ice concentration, and ecosystem. The mooring provides time series of physical and biogeochemical properties at a location that is critical for understanding the fate of Pacific water in the Arctic and its impacts. Measurements of mass, heat, and freshwater fluxes, as well as transport of biogenic material and upwelling and downwelling events are also documented and quantified from the mooring data. This allows for identification of causal relationships between the physical environment and the ecosystem, ranging from nutrient dynamics to higher trophic levels.
As one node in a greater network of moored observatories that monitor Alaska’s large marine ecosystems, the University of Alaska Fairbanks (UAF) Chukchi Ecosystem Observatory (CEO) program maintains a cluster of highly instrumented subsurface moorings on the Northeast Chukchi continental shelf (Hauri et al., 2018). Electronic, optical, and acoustic data loggers record measurements that characterize the physical environment (temperature, salinity, pressure, currents, waves, ice draft, irradiance, optical backscatter), the chemical environment (NO3, colored dissolved organic matter, dissolved oxygen, pH, pCO2), phytoplankton standing stock (chlorophyll a fluorescence), zooplankton and fish densities (acoustic backscatter), and marine mammal presence (underwater sound). An autonomous time-series sampler collects discrete water samples for subsequent laboratory analyses of eDNA and inorganic nutrients (NO3, NO2, NH4, SiO3, PO4); time-series sediment traps collect zooplankton and meroplankton, along with sinking particulate matter for quantification of fluxes of phytoplankton species, total particulate matter (TPM), particulate organic carbon (POC), and zooplankton fecal pellets (Lalande et al., 2021). A time lapse camera system planned for deployment in 2022 will capture a photographic history of the seafloor, fishes, and epifauna. These co-located observations thereby provide views into the year-round functioning of the Chukchi shelf ecosystem across all trophic levels and spanning the benthic, pelagic, and sympagic (ice) realms.
By deploying passive acoustic sensors on gliders and oceanographic moorings instrumented with other sensors, the relationship between top trophic level marine mammal occurrence and their environment can be elucidated (Stafford et al., 2021). The University of Washington has deployed acoustic sensors quasi-continuously year-round on oceanographic moorings since 2008 in the Bering Strait, Beaufort Sea, and Northern Chukchi Sea; since 2016 at the Chukchi Ecosystem Observatory; and since 2013 on gliders deployed from the Bering Strait moorings cruise for annual transits of the Chukchi shelf from Bering Strait to Barrow Canyon (Baumgartner et al., 2014). The AFSC Marine Mammal Laboratory has maintained approximately 20 subsurface passive acoustic recorder moorings that extend from the Aleutian Islands to the central Beaufort Sea and the Northern Chukchi Sea as part of the Arctic Long-Term Integrated Mooring Array (ALTIMA). ALTIMA moorings have been deployed annually for over a decade, with most co-located with biophysical instrumentation (see EcoFOCI below) and at the five original DBO sites.
For both passive acoustic programs, acoustic presence is determined for Arctic and subarctic marine mammal species including baleen whales, odontocetes, and pinnipeds. Because these species represent a wide variety of feeding guilds, from specialists to generalists, from planktivores to piscivores, and from obligate to facultative benthic feeders, these data are ideal for examining the consequences of change in many different bottom-up processes. Passive acoustic data have provided novel information on the migratory timing and presence of Arctic and subarctic species. For instance, bowhead and beluga whales have recently delayed their fall migrations out of the high Arctic (Hauser et al., 2017; Stafford et al., 2021), subarctic fin and killer whales are moving north and spending more time in an ice-free Chukchi Sea (Stafford, 2019; Escajeda et al., 2020), and critically endangered North Pacific right whales are being detected further north in the Bering Sea (Wright et al., 2019). The data provide baseline noise levels for evaluating acoustic environment changes caused by increased industrial and vessel traffic presence and climate change-induced extension of the open-water season (Wright et al., 2018).
NOAA’s Ecosystems and Fisheries-Oceanography Coordinated Investigations (EcoFOCI) program assesses the ecosystems of the North Pacific Ocean, the Bering Sea, and the US Arctic to improve understanding of ecosystem dynamics and apply that understanding to the management of living marine resources (Tabisola et al., 2021). EcoFOCI scientists integrate field, laboratory, and modeling studies to determine how variability in biological and physical factors, including climate, influence Alaska’s large marine ecosystems. Long-term moored observatories are maintained at five sites in the Bering Sea (Figure 2b) and seven sites in the Chukchi Sea. These measurements usually include ice draft, currents, temperature, salinity, chlorophyll fluorescence, oxygen, nitrate, and passive acoustics to detect marine mammals. Select sites also include sediment traps that collect sinking organic and inorganic particles; water samplers for isotopes, nutrients, eDNA, and phytoplankton speciation; and instruments that measure pCO2, colored dissolved organic matter, primary production via oxygen, and gas ratios. Technological advances are improving our ability to observe the Arctic. For instance, new moorings have a surface component that is programmed to sink with sea ice arrival. During the following spring, instruments detect sea ice retreat, and at the appropriate time, the surface component re-floats and resumes sampling. Other innovations include low-cost air-deployed coastal profiling floats, under-ice pop-up floats, in situ microbial incubation systems, and imaging systems with artificial intelligence to estimate, identify, and determine the abundance of phytoplankton, zooplankton, and fish.
EcoFOCI moored observations are closely linked to annual research cruises that assess the rapidly changing Bering Sea and the US Arctic. Three of the moorings are located in DBO regions, while others are associated with long-term NOAA cruise transects and seabird sampling, including the Bering Sea 70 m isobath transect and transects across Unimak Pass and at Icy Cape. Sampling on these transects includes CTDs; collection of discrete water samples for chlorophyll, nutrients, oxygen and eDNA; and zooplankton nets; visual observations detect marine mammals and birds. The hydrographic transects are conducted once or twice a year, and data from the moorings place them within a longer temporal context. The data validate and inform regional ecosystem models and play an important role in understanding ecosystem dynamics, especially in the context of climate and ocean changes (Hunt et al., 2011; Stabeno and Bell, 2019; Stabeno et al., 2020).
Final Remarks
The efforts represented by this collection of collaborative long-term monitoring programs enable a biologically focused scientific foundation for understanding how the Alaskan Arctic marine ecosystem is changing as water temperatures warm and sea ice declines. We expect these data will continually improve the ability of resource management and US mission-oriented agencies (e.g., NOAA, Bureau of Ocean Energy Management) to refine actions that impact marine resources, local communities, and biological systems.
Although organizationally separate, there exist many formal and informal linkages between the programs described herein. Oceanographic moorings carry passive acoustic recorders, seabird observers join at-sea surveys, and social media postings from community-based programs document local observations that inform research scientists. Biogeochemical time-series data from field studies help constrain and validate the functioning of Earth system models. Such partnerships encourage cross-program, interdisciplinary collaborations that help scientists leverage the monitoring data into applications to emerging issues and analyses that were not necessarily identified at the start of each monitoring program. For example, mooring, shipboard hydrography, plankton net, fisheries trawl, and seabird data together helped Sigler et al. (2011) refine our understanding of PAR biogeographical provinces and their relations to the physical environment.
In addition to the extensive efforts described here, numerous additional ongoing long-term monitoring programs include, but are not limited to: study of black guillemots at Cooper Island (Divoky et al., 2021); benthic studies of the Stefansson Sound Boulder Patch kelp community (Wilce and Dunton, 2014); monitoring of seabird mortality by the Coastal Observation and Seabird Survey Team (COASST; Parrish et al., 2017); USFWS Alaska Maritime National Wildlife Refuge seabird colony monitoring; US Geological Survey polar bear surveys; State of Alaska Norton Sound bottom trawl surveys (Hamazaki et al., 2005) and ice seal data sets (Crawford et al., 2015); assessments of harmful algal blooms (with some undertaken as part of the DBO effort; Anderson et al., 2021); community-based monitoring of coastal erosion; aerial surveys of marine mammal populations; NOAA’s Ocean Noise Reference Station Network; Korean surveys on the icebreaker R/V Araon; Chinese observations from the icebreaking R/V Xue Long; Russian fishery surveys in the Russian Federation exclusive economic zone; the North Slope Borough Division of Wildlife Management bowhead whale survey and other studies; investigations of bowhead whale prey (Ashjian et al., 2021); studies conducted by Kawerak and other regional nonprofit corporations; and efforts organized by environmental coordinators in most coastal villages. The Local Environmental Observer (LEO) program is a citizen observation network with participants distributed throughout Alaska (Brubaker et al., 2013), as is the Indigenous Sentinels Network (Divine and Robson, 2020). Satellite-based sensors provide additional monitoring context with decades of ice cover and ocean color measurements (e.g., Frey et al., 2015).
All of the projects listed above and in Table 1 deal with the inevitable challenges and uncertainties in funding availability, the vagaries of weather and sea ice, and the surprises that arise while doing Arctic fieldwork. This paper serves as a testament to the perseverance needed to accomplish reliable observations over extended periods of time, to the societal importance ascribed to maintaining such efforts, and to the benefits of inter-program collaborations. Over time, the value of long-term monitoring data only increases as new questions emerge that can be assessed with the benefit of existing monitoring data, and especially when new data applications arise through synergy of independently operating efforts.
Acknowledgments
We thank the countless field crew members, vessel operators, funding agencies, and local collaborators who have contributed to all of the efforts described in this paper. We thank Justin Crawford for assistance with seal data and Dan Cushing with seabird data. We thank Steve Okkonen and one anonymous reviewer for helpful comments that improved the manuscript. The scientific results and conclusions, as well as any views or opinions expressed herein, are those of the author(s) and do not necessarily reflect those of NOAA or the Department of Commerce. Reference to trade names does not imply endorsement by the National Marine Fisheries Service or NOAA. Funding sources include the following: ALTIMA: BOEM M09PG00016, M12PG00021, and M13PG00026; AMBON: NOPP-NA14NOS0120158 and NOPP-NA19NOS0120198; Bering Strait moorings: NSF-OPP-AON-PLR-1758565, NSF-OPP-PLR-1107106; BLE-LTER: NSF-OPP-1656026; CEO: NPRB-L36, ONR N000141712274 and N000142012413; DBO: NSF-AON-1917469 and NOAA-ARP CINAR-22309.07; HFR, AOOS Arctic glider, and Passive Acoustics at CEO and Bering Strait: NA16NOS0120027; WABC: NSF-OPP-1733564. JAMSTEC: partial support by ArCS Project JPMXD1300000000 and ArCS II Project JPMXD1420318865; Seabird surveys: BOEM M17PG00017, M17PG00039, and M10PG00050, and NPRB grants 637, B64, and B67. This publication was partially funded by the Cooperative Institute for Climate, Ocean, & Ecosystem Studies (CICOES) under NOAA Cooperative Agreement NA20OAR4320271, and represents contribution 2021-1163 to CICOES, EcoFOCI-1026, and 5315 to PMEL. This is NPRB publication ArcticIERP-43.