Background and Setting
The northern California Current System is known for high rates of primary productivity (Hickey and Banas, 2008) and valued finfish and shellfish resources. Coastal ecosystems in the Pacific Northwest have exhibited intensifying hypoxia, ocean acidification, and marine heatwaves over recent decades (Chan et al., 2008; Feely et al., 2008, 2016; Peterson et al., 2013; Koehlinger et al., 2023). Oxygen levels decrease in warming waters with increasing stratification (e.g., Schmidtko et al., 2017), while ocean uptake of excess carbon dioxide (CO2) in the atmosphere from human activities drives an increase in ocean acidity (decrease in pH) and a decrease in calcium carbonate saturation states (Ω) (Feely et al., 2004, and 2023, in this issue). Marine heatwaves and hypoxia have caused mass marine mortality events since 2000 (e.g., Grantham et al., 2004; Piatt et al., 2020), but recent research shows that acidified conditions are more pervasive and persistent in Washington’s marine waters than low oxygen events (recent work of author Alin and colleagues).
The northwest coast of Washington state has been home for millennia to four Coastal Treaty Tribes, the Hoh Tribe, Makah Tribe, and Quileute Tribe, and the Quinault Indian Nation, who all rely extensively on marine resources for subsistence, cultural, and economic well-being (Figure 1). All four sovereign tribal nations hold unique rights to ocean resources that were reserved in perpetuity through treaties signed with the US government in the 1850s. Today, fishery resources on the Olympic Coast are co-managed primarily by the tribes and the state of Washington, including within NOAA’s Olympic Coast National Marine Sanctuary (OCNMS), which overlaps most of the tribal treaty fishing areas. To understand and inform tribal, state, and federal managers about the exposure of marine species and ecosystems to multiple marine stressors, the NOAA Pacific Marine Environmental Laboratory (PMEL) has partnered with OCNMS and the University of Washington since 2009 to develop and analyze ocean time series and numerical models for ocean acidification in Washington’s coastal waters. In 2017, NOAA’s Ocean Acidification Program funded this team to partner with tribal representatives and conduct a regional assessment of the vulnerability of the Coastal Treaty Tribes of Washington to the impacts of ocean acidification on marine species important to the tribes. Here, we present a novel analysis of ocean acidification change estimates from the pre-industrial era (when atmospheric CO2 was 280 parts per million) to the year 2100 and use Dungeness crab as a case study for understanding the relevance of rapidly changing coastal ocean conditions to coastal communities in Washington.
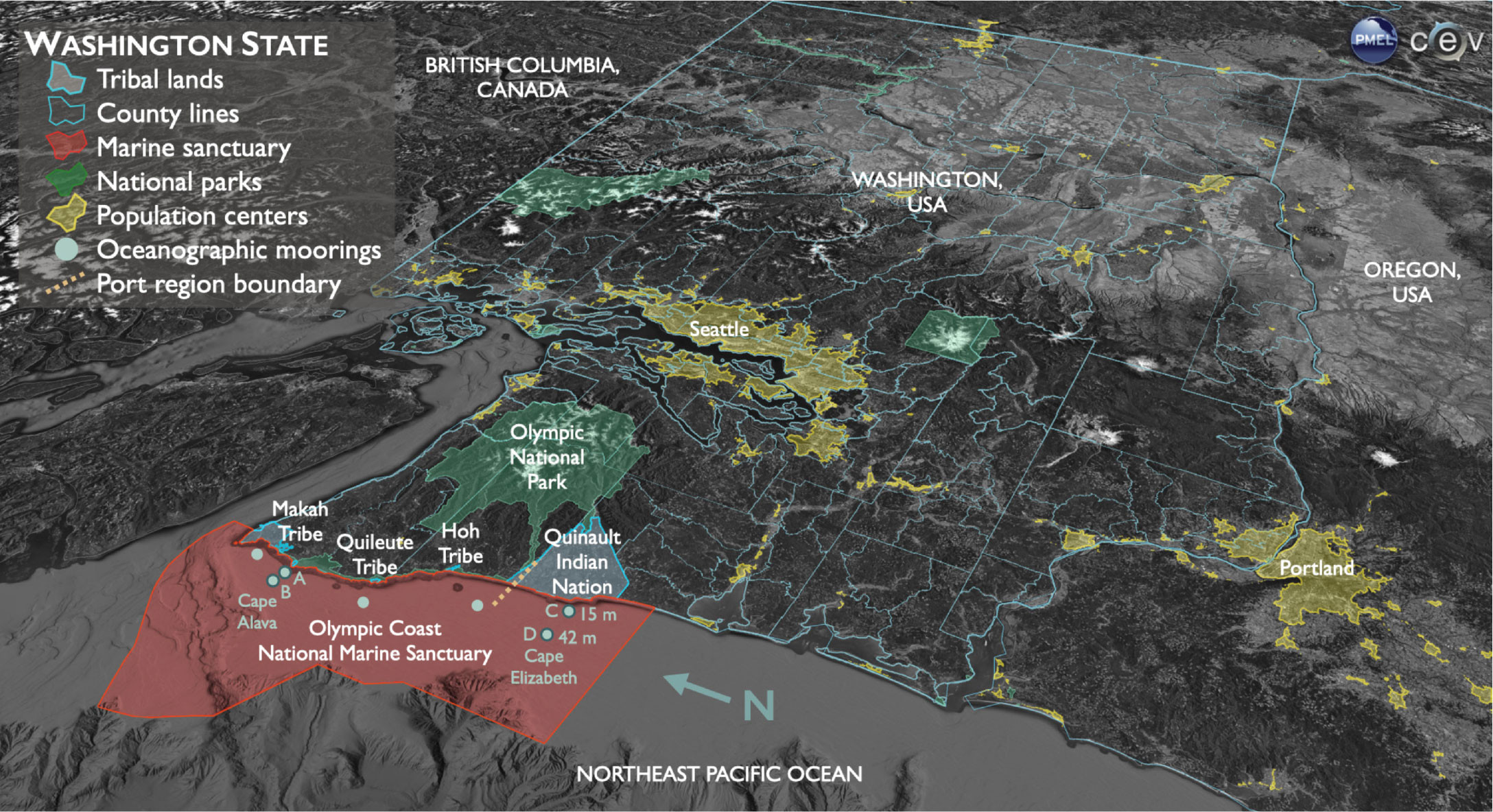
FIGURE 1. The Olympic Coast is at the northwest corner of Washington state, with the Olympic National Park (green) and the Olympic Mountains onshore and upland and the Northeast Pacific Ocean offshore. Treaty areas on land of the four Coastal Treaty Tribes—the Hoh Tribe, Makah Tribe, and Quileute Tribe, and the Quinault Indian Nation—are outlined in cyan. Locations of the seven near-bottom Olympic Coast National Marine Sanctuary moorings equipped with temperature, salinity, and oxygen sensors are shown in teal, with the two pairs of moorings highlighted in this paper outlined in a darker shade. The northern moorings are offshore of Cape Alava (a and b in Figures 3 and 4), and the southern moorings are offshore of Cape Elizabeth (c and d in Figures 3 and 4). The approximate boundary between northern and southern Washington port regions used by Hodgson et al. (2018) is indicated by a dashed line (goldenrod). Image created by Hunter Hadaway. > High res figure
|
Dungeness Crab Importance to Washington Fisheries and Their Ocean Acidification Vulnerability
Dungeness crab (Metacarcinus magister) has been the most valuable commercial fishery on the Washington coast for decades (Rasmuson, 2013). It has been sustainably fished by tribal and state-managed fisheries for half a century, despite the high annual catch rate of legal-sized males (Richerson et al., 2020). However, ecosystem modeling studies suggest large declines in catch and resulting economic impacts to the Dungeness crab fishery from ocean acidification as soon as 2060–2070 under the high-emissions RCP 8.5 CO2 scenario, with particular consequences for Washington ports (Marshall et al., 2017; Hodgson et al., 2018). Coupled social-ecological work indicates the importance of small-scale human dependencies on marine resources (Ekstrom et al., 2015). These studies do not explicitly address tribal communities’ marine resource dependency, but the results underscore the relevance of localized oceanographic conditions offshore to the onshore well-being of place-based communities like the Coastal Treaty Tribes of Washington.
Numerous studies have evaluated Dungeness crab sensitivity to acidified conditions across life stages and in both laboratory and field settings (Figure 2). Experiments reflecting projected future pH/CO2 conditions for Washington marine environments have shown delayed hatching and larval development in the earliest nearshore (eggs) and pelagic (zoea) Dungeness crab life stages, with higher larval mortality (Miller et al., 2016). In contrast, late-stage pelagic megalopae and benthic juveniles exhibited higher survival, faster transitions among life stages, smaller sizes, and higher respiration rates under elevated CO2 experimental conditions (McElhany et al., 2022). PMEL-led West Coast Ocean Acidification (WCOA) cruises revealed in situ correlations between depth gradients of calcite saturation states (Ωcalc) and exoskeleton dissolution in megalopae, with implications for damage to mechanoreceptors that serve important sensory and behavioral functions (Bednaršek et al., 2020). Exoskeleton density in laboratory-reared zoea and field-caught megalopae also correlated with acidification metrics, which may reflect energy requirements for active ion pumping to attain calcite supersaturation levels needed for calcification (Saenger et al., 2023).
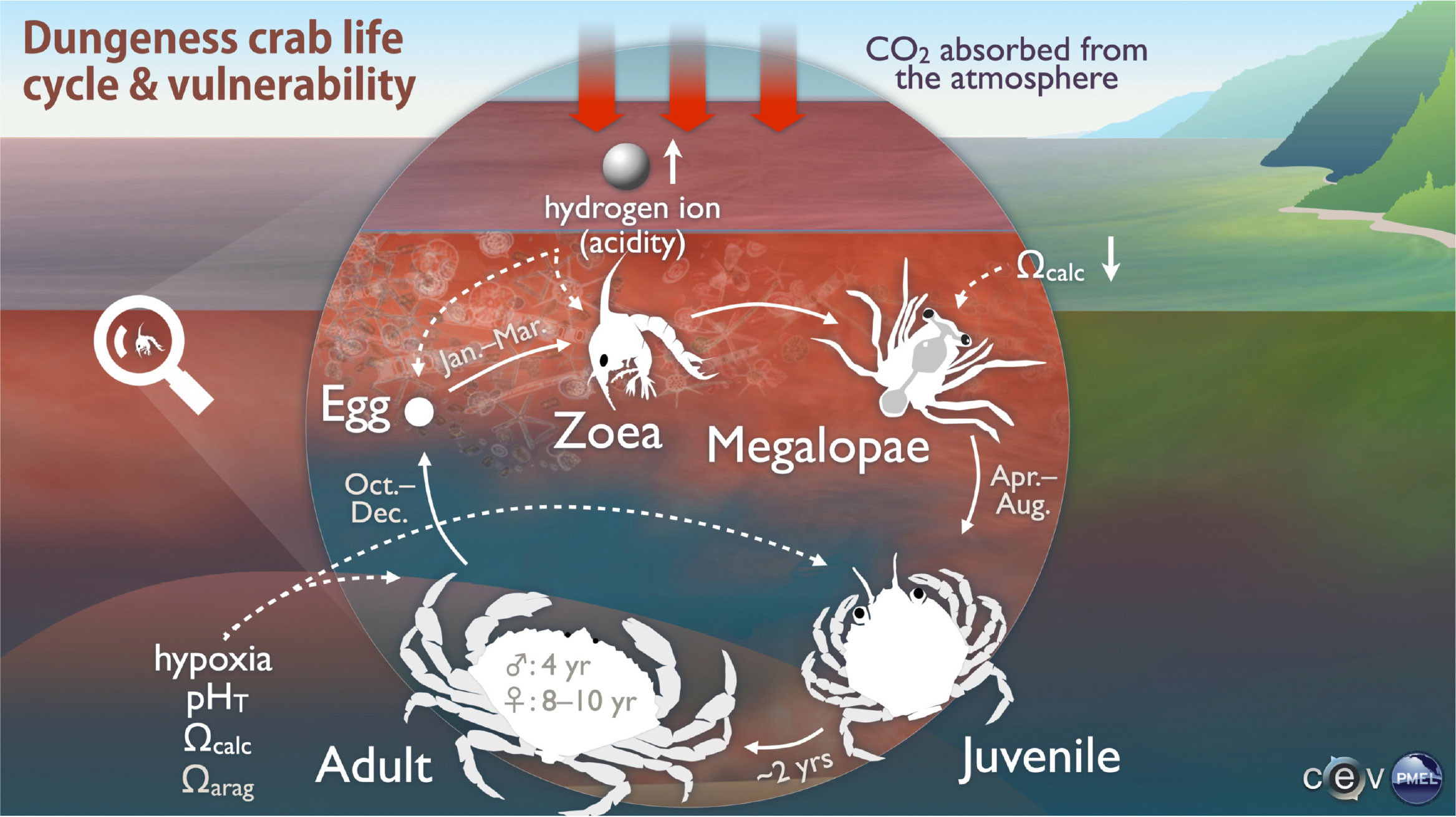
FIGURE 2. The Dungeness crab life cycle and vulnerability to ocean acidification and hypoxia by life stage. Dungeness crab eggs are brooded by adult females in nearshore environments until they hatch, while larval phases—zoea and megalopae—are pelagic, and juvenile and adult life stages occupy benthic habitats, with timing shown in the figure (Pauley et al., 1989; Rasmuson, 2013; Berger et al., 2021). Ocean acidification stressors are related to their respective life stages by arrows, with indirect effects via prey species postulated for aragonite saturation state (Ωarag) and direct effects for acidity and Ωcalc. Image created by Hunter Hadaway. > High res figure
|
An assessment of Dungeness crab vulnerability to ocean acidification across all life stages showed that while consequences of exposure to acidified conditions are more severe at earlier life stages, integrated vulnerability is dominated by the longer duration of exposure to more acidified conditions during later, benthic life stages that contribute strongly to population growth (Hodgson et al., 2016). Numerical models developed by PMEL partners at the University of Washington and the University of Connecticut suggest that, while adult exposure to hypoxic, acidified conditions occurs during the upwelling season today, exposure to harmful acidification is projected to be year-round for all life stages by 2100 under the RCP 8.5 scenario, indicating that the present-day wintertime reprieve from acidified conditions will no longer occur (Berger et al., 2021). Scientists who provide ongoing seasonal forecasts of coastal ocean conditions aim to illuminate relationships between pelagic life stage exposure and subsequent fishery recruitment and to co-develop tools with tribal and state fisheries managers to better anticipate annual Dungeness crab catches (Norton et al., 2020, 2023; Siedlecki et al., 2023, in this issue).
Time-Series and Model Estimates of Past, Present, and Future Ocean Acidification Status
As part of its management responsibility, OCNMS has deployed time-series moorings during upwelling seasons (April–September) since 2006 to monitor benthic hypoxia that develops seasonally at shallow to mid-shelf depths. A subset of these moorings has sensors that measure oxygen (O2), temperature (T), and salinity (S) roughly one meter above the seafloor from approximately April to October each year. The oceanographic moorings are deployed from north to south within the sanctuary (Figure 1) and provide rich information on Olympic Coast spatial variability, with implications for exposure of the marine resources that the Coastal Treaty Tribes depend on to multiple stressors. We focus here on examining shallow (15 m depth) and mid-shelf (42 m depth) results for northern and southern mooring locations (off of Cape Alava and Cape Elizabeth, respectively), which consistently manifest different levels of seasonal acidification and hypoxia (Office of National Marine Sanctuaries, 2022).
We used a Washington coast subset of high-quality physical and biogeochemical measurements collected on 2007–2016 WCOA cruises as calibration data for developing empirical relationships in order to estimate present-day dissolved inorganic carbon (DIC) and total alkalinity (TA), using T, S, and O2 data as proxy variables (following the approach of Alin et al., 2012, and references therein). The calibration data set consisted of 357 TA and 362 DIC samples, with complete T, S, and O2 data available for each sample, collected at depths of 10–150 dbar at 46 stations over the continental shelf and encompassing the OCNMS moorings (47°N–48.5°N). Using the multiple linear regressions (MLRs) with the lowest root mean squared errors, we translated the 2006–2019 oceanographic mooring time-series data into estimated DIC and TA time series for the four sites.
We subsequently estimated the anthropogenic carbon (Canth) content for each time-series measurement using T, S, and year using MLRs developed from multi-decadal Global Ocean Ship-based Hydrographic Investigations Program (GO-SHIP) carbon inventories based on Pacific Ocean surveys (Feely et al., 2016). To generate pre-industrial estimates of carbonate chemistry, we subtracted each Canth value from its respective estimated DIC value. We also generated site-specific linear regressions of all 2006–2019 Canth values to estimate rates of increase at each mooring site and used these local rates of Canth increase to project DIC levels in 2030. To ensure that present-day estimates were not biased by the timing of data gaps across years and sites, we adjusted all present-day DIC estimates to a 2010 index year using the same approach.
We then used six-hourly mooring T and S data, TA estimates from MLRs, and the DIC estimates for the pre-industrial era, the present (2010), and the near future (2030) to calculate other carbonate system parameters, specifically pH on the total scale (pHT) and saturation states for aragonite and calcite forms of calcium carbonate (Ωarag and Ωcalc), using R package seacarb (Gattuso et al., 2022). Monthly mean values for the pre-industrial era, 2010, and 2030 represent averages of all carbonate chemistry estimates made within that month across the full 6–14 years of observations available from each mooring location (i.e., minus data gaps; Figures 3 and 4). Thus, these estimates incorporate the interannual variability recorded in the time series.
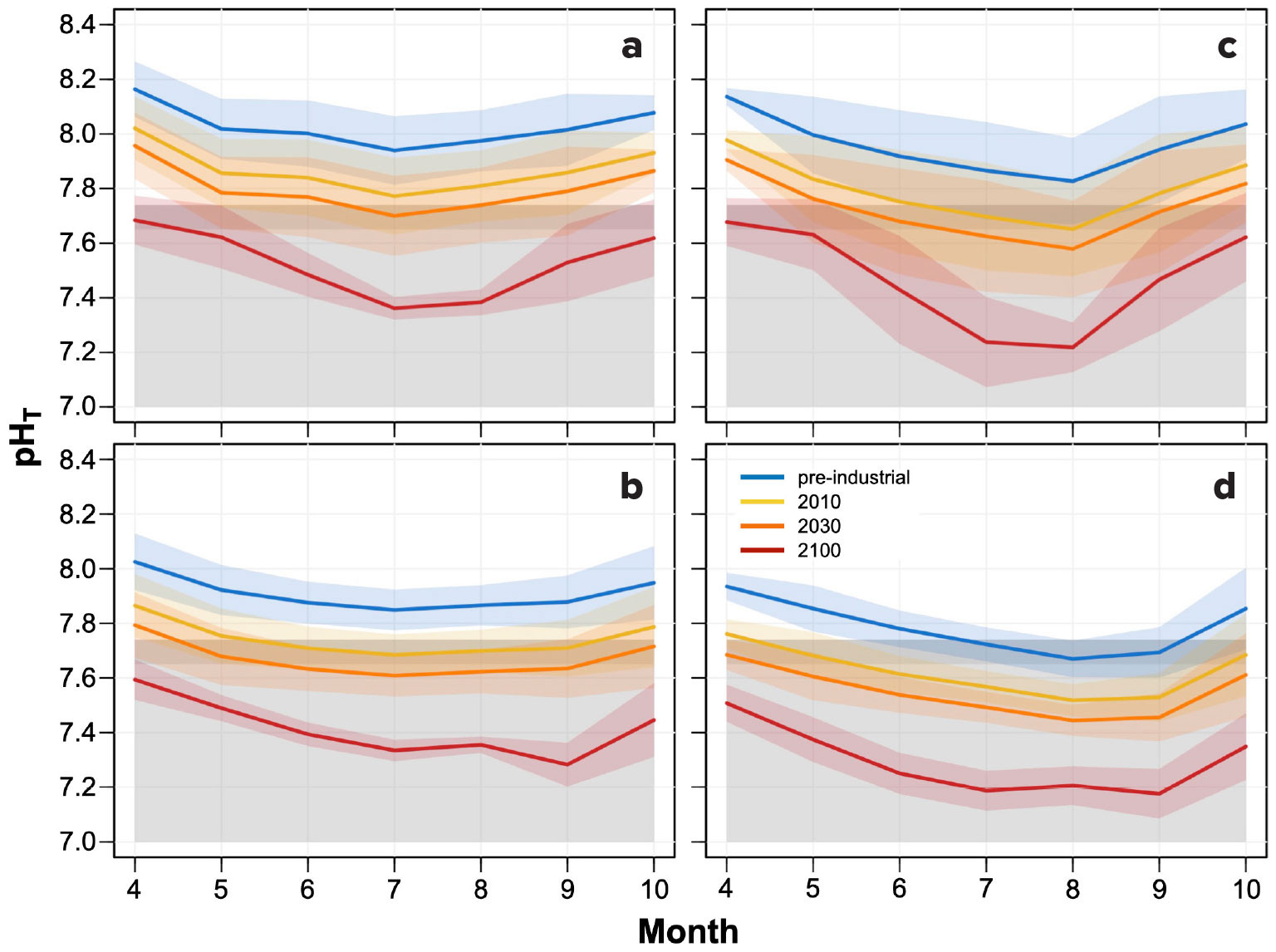
FIGURE 3. Monthly average pH levels (on the total scale, pHT) at the four mooring sites: (a) the northern shallow site (Cape Alava, 15 m depth), (b) the northern deep site (Cape Alava, 42 m), (c) the southern shallow site (Cape Elizabeth, 15 m), and (d) the southern deep site (Cape Elizabeth, 42 m). Shaded areas reflect one standard deviation of the mean, representing variation around average conditions. Gray shading between pHT 7.65 and 7.74 indicates the sensitivity window in which adult Dungeness crab thresholds may begin to be exceeded, with lighter shading below this band reflecting conditions that have exceeded this window. > High res figure
|
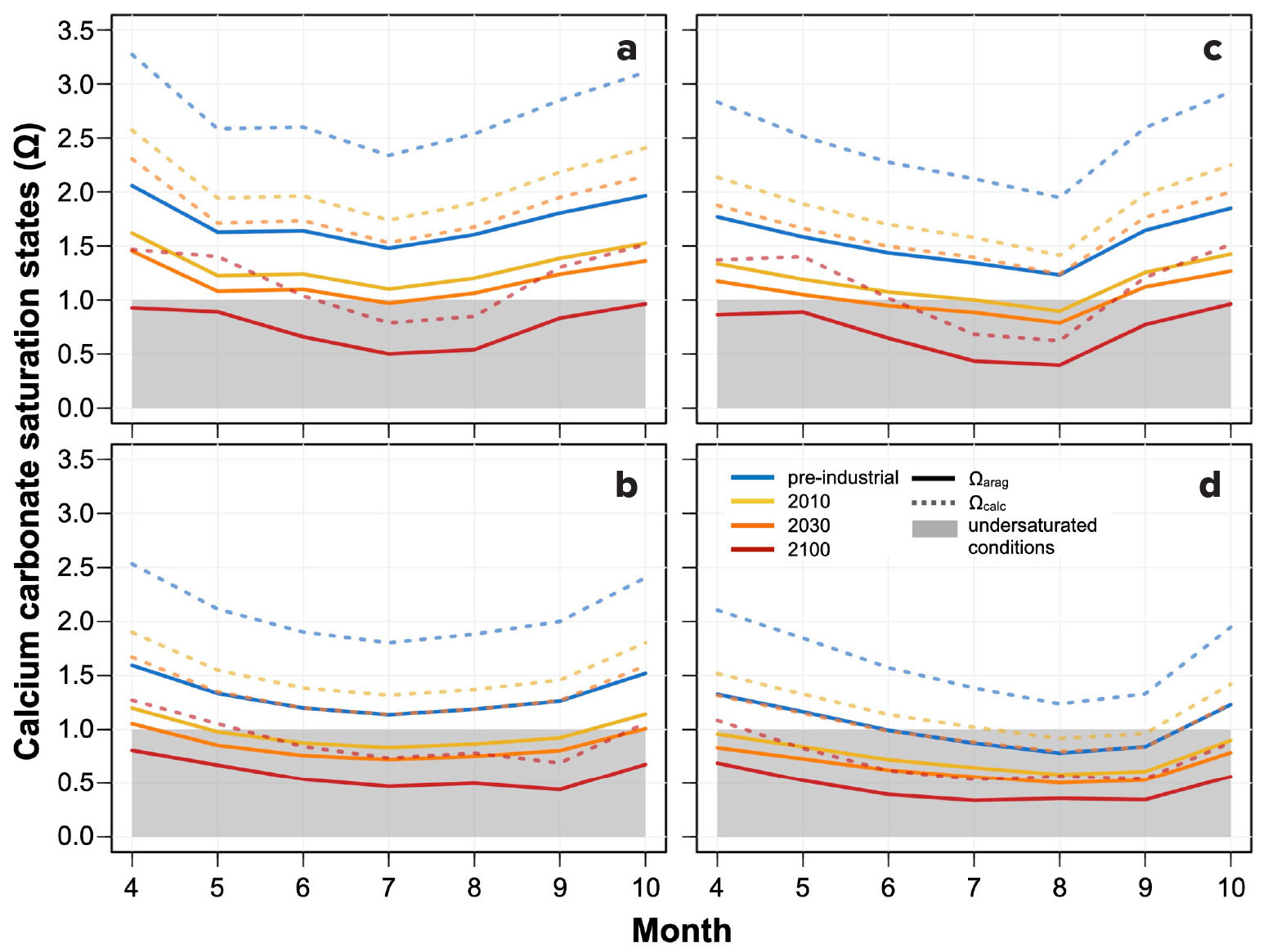
FIGURE 4. Monthly average values of aragonite (Ωarag, solid lines) and calcite (Ωcalc, dotted lines) saturation states. Gray shading below Ω = 1 reflects saturation states below thermodynamic equilibrium. Organization of panels and colors are the same as in Figure 3. > High res figure
|
Our estimates of pH and Ω change by 2030 are highly uncertain, because they simply extrapolate recent rates of Canth increase at each location into the future. These estimates are governed by modern oceanographic conditions and do not reflect potential future redistributions of water masses, which could have large impacts on coastal and benthic seawater chemistry. However, if oceanographic water masses and processes remain similar to conditions observed during the 2006–2019 time-series by 2030, these estimates would provide conservative estimates of carbonate chemistry change, as they do not account for increasing rates of Canth accumulation in subsurface water masses that are presently in transit to Northeast Pacific upwelling regions and will upwell in this region with a higher Canth burden by 2030 (e.g., Franco et al., 2021).
Projected monthly pHT, Ωarag, and Ωcalc averages and variations for the end of the century were generated by the Cascadia model, which is a high-resolution (1.5 km) three-dimensional coupled physical and biogeochemical Regional Ocean Modeling System (ROMS) model (Berger et al., 2021; Siedlecki et al., 2021). The more sophisticated and complex Cascadia projection does account for changes in circulation, river runoff, and oceanographic conditions in a way that our simple near-future projection of Canth cannot.
Pre-industrial Era to End-of-Century (2100) Estimates of Acidification Conditions that Exceed Dungeness Crab Thresholds
For this case study, we focus on how pHT, Ωarag, and Ωcalc changes through time have altered the upwelling season exposure of juvenile and adult Dungeness crab inhabiting Olympic Coast benthic habitats. Synthesis of decapod sensitivity thresholds, including studies on Dungeness crab, indicates negative effects on adult respiration and hemolymph pH with exposure to environmental pHT levels of 7.70–7.74 for 13–21 days (Bednaršek et al., 2021). Dungeness crab-specific ocean acidification vulnerability assessments have used pH = 7.65 as a threshold for negative consequences across life stages (Hodgson et al., 2016; Berger et al., 2021). We thus compare observed monthly average pHT conditions across time to a 7.65–7.74 “sensitivity window.” We also present Ωarag and Ωcalc changes through time because projected Dungeness crab population impacts may be driven by declines in aragonitic bivalve prey species (Marshall et al., 2017) or relationships between Ωcalc gradients and megalopal exoskeleton damage (Bednaršek et al., 2020). However, because specific thresholds are not yet known, we present these results relative to their thermodynamic saturation thresholds (Ω = 1).
Monthly pHT averages and variation around the mean across the mooring sites reveal substantial spatial differences (Figure 3). During all time periods (pre-industrial era [PI], 2010, 2030, 2100), shallower sites have higher mean pHT than deeper sites on each transect, and northern sites have higher mean pHT than southern sites at each depth. Southern transect moorings show a more pronounced progression toward lower pHT during the upwelling season than the northern sites, reflecting seasonal consumption of O2 and increase in CO2 related to localized retention of bottom waters on the shelf near Cape Elizabeth (Hickey and Banas, 2008; Connolly et al., 2010; Peterson et al., 2013; Siedlecki et al., 2015). Consequently, acidification metrics—pHT and Ω—reach more extreme levels on the southern transect by the late upwelling season (i.e., August–September) than in northern waters (Figures 3 and 4).
Temporal change varied substantially across sites as well. Only the deep southern mooring showed average monthly conditions that crossed the upper Dungeness crab pHT threshold during the pre-industrial era (July–September; Figure 3). In contrast, all sites except the shallow northern mooring dipped into or below the same sensitivity window by 2010. By 2030, even the shallow northern site may have average monthly pHT conditions within the threshold range during July, while average conditions below this threshold may prevail at the other sites for up to six months each year. By 2100, average April conditions at the shallow sites are projected to fall within the sensitivity window, while all other months and sites are expected to fall below it.
Onset timing, duration, and severity of acidified conditions likely also matter for Dungeness crab populations. The lowest PI–2030 monthly pHT means at shallow sites occur during July in the north and August in the south, broadening to July–August by 2100 at both sites. Minimum monthly mean 2100 pHT values are projected to fall to 7.4 and 7.2 in the north and south, respectively, compared to estimated PI minima of >7.9 and >7.8. At deeper moorings, minimum PI–2030 pHT estimates also occurred during July in the north and August–September in the south. By 2100, lows are projected to occur in September at both deep locations, with monthly means of 7.3 and 7.2 in the north and south, compared to PI estimates of >7.8 and ~7.65, respectively. Across sites, monthly means cross into the Dungeness crab sensitivity window earlier in the upwelling season as time progresses. Relative to PI conditions, overall declines in monthly mean pHT averaged 0.16 by 2010, 0.24 by 2030, and 0.50 by 2100. However, comparing changes in pHT through time provides an inaccurate measure of acidity change when the initial pHT levels vary across sites, as they do here, because the logarithmic scale of pH provides relative rather than absolute measures of [H+] change (Fassbender et al., 2021). These pHT changes equate to average increases in upwelling season acidity ([H+]) since the PI of 44%–46% across sites by 2010, 69%–74% by 2030, and 213%–220% by 2100, with the smallest change through time at the deep southern site where conditions are consistently the most acidified. Compared to an annually averaged pHT decline of 0.31 on the northern California Current System shelf across the twenty-first century (Siedlecki et al., 2021), upwelling season monthly averages are projected to decrease by 0.20 to 0.46 across 2010–2100, highlighting the relevance of localized rates of change to benthic species.
The bottom water pHT seasonal amplitude for the upwelling season—the difference between the lowest and highest monthly means—spanned 0.18–0.31 in the pre-industrial era, 0.18–0.33 in 2010 and 2030, and 0.31–0.46 in 2100, with the smallest seasonal amplitudes at the northern deep site and the largest at the shallow southern mooring. Whereas global surface ocean future simulations project narrowed seasonal cycles (Kwiatkowski and Orr, 2018), our end-of-century projection suggests that pHT seasonal amplitude may expand by 2100 in these coastal subsurface environments, although projected change appears minimal from the PI to 2030. In order to understand the magnitude of change in the seasonal amplitude of acidity, we also compared [H+] through time. [H+] seasonal amplitude estimates expanded progressively across these benthic sites from the PI to 2100, with 260%–385% amplification during 2010–2100 compared to an 81% ± 16% amplification in a contemporaneous global surface ocean simulation (cf. Kwiatkowski and Orr, 2018).
While specific Ω thresholds are not known for Dungeness crab or their dominant prey, the correlation between exoskeleton dissolution and Ωcalc gradients (Bednaršek et al., 2020) and the projected fishery impacts driven by declines in Ωarag-sensitive bivalve prey species (Marshall et al., 2017) suggest that future Ω changes in the region will also be an important consideration for Dungeness crab fishery managers. Seasonal dynamics for Ω show the same patterns across sites as pH, with a seasonal progression toward lower Ω conditions at southern sites and lower values at deep moorings on both transects across all time periods (Figure 4). During the PI, only the deep southern mooring registered estimated monthly mean Ωarag undersaturation, which may be harmful to Dungeness crab bivalve prey species, but by 2100 all sites and depths are projected to show monthly mean Ωarag undersaturation, with the lowest monthly Ωarag means being 0.44–0.50 at northern moorings and 0.34–0.40 at southern moorings. Between the PI and 2010, we estimate that upwelling season monthly mean Ωarag values decreased by an average of 0.28–0.41 across these mooring locations, with total average declines of 0.38–0.56 estimated by 2030 and 0.57–0.98 by 2100. Benthic Ωcalc values are related to Ωcalc depth gradients that correlate with present-day megalopae dissolution (Bednaršek et al., 2020). A striking observation is that two to six months of mean Ωcalc undersaturation are projected for all sites by 2100, with potentially four months of Ωcalc undersaturation at the deep southern site by 2030. The lowest monthly Ωcalc means are projected to be 0.69–0.79 in the north and 0.53–0.62 in the south in 2100. Monthly Ωcalc means have declined an estimated 0.45–0.66 from the PI to 2010, and may decline a total of 0.60–0.89 by 2030 and 0.91–1.56 by 2100. Unlike pHT, the average twenty-first century decline in upwelling season monthly Ω means across sites (Ωarag: –0.29 to –0.57; Ωcalc: –0.47 to –0.91) were mostly smaller than the annually averaged declines (Ωarag: –0.50; Ωcalc: –0.79; Siedlecki et al., 2021). However, similarly to pH, the smaller average monthly changes are anticipated to occur at the sites that are already more acidified, with deep and southern moorings showing smaller projected changes than their shallow and northern counterparts. Seasonal Ωarag (Ωcalc) amplitudes also progressively decrease as mean saturation states decline across time intervals at all locations from 0.46–0.62 (0.73–0.98) in the PI to 0.33–0.57 (0.52–0.89) in 2100. Global end-of-century models suggest neither strong amplification nor attenuation of Ωarag seasonal amplitudes in this region (Kwiatkowski and Orr, 2018), so these findings suggest substantially different futures in these dynamic and spatially variable benthic coastal environments.
While monthly means provide a useful characterization of the trajectory of long-term acidification trends, organisms are also harmed by shorter-term exposure to extreme conditions. Estimates of the frequency at which daily average acidification conditions fell below the pHT and Ω thresholds during the upwelling season vary widely across the moorings and through time. At the shallow northern site, Ωarag (Ωcalc) values fell below their saturation thresholds <1% (0%) of time during the PI, while undersaturated Ω values are projected to occur 23% (1.5%) of the time by 2030. In the PI, daily average pHT values never exceeded the 7.65 threshold, while in 2030, projected upwelling season exceedance is estimated at 11%. At the deep southern mooring, daily PI Ωarag (Ωcalc) means were undersaturated for 71% (<1%) of the upwelling season, compared to 98% (73%) of the time during 2030. Comparable frequencies for pHT are estimated at 18% in the PI vs. 93% by 2030. Finally, minimum daily means for Ωarag, Ωcalc, and pHT during the PI were estimated as 0.89, 1.41, and 7.73 at the shallow northern site (0.56, 0.88, and 7.52 at the deep southern site), compared to 2030 projections of 0.55, 0.87, and 7.48 (0.36, 0.57, and 7.30), respectively. These results suggest that the most extreme present-day acidification conditions at the shallow northern site resemble those from the PI at the deep southern mooring location.
“Differences between northern and southern Olympic Coast acidification mean conditions and seasonality across time suggest an opportunity to use this region as a testbed for understanding how small-scale regional variations in ocean conditions may affect realized future climate impacts to fisheries.”
|
Implications for Olympic Coast Water Quality and Fishery Management
End-of-century multiple stressor projections suggest that Dungeness crab population vulnerability will be dominated by upwelling season adult exposure to hypoxia (Berger et al., 2021). Fisheries research on crab spatial distributions in the nearby Salish Sea showed that Dungeness crab migrated to shallower waters during hypoxic conditions rather than moving laterally to less hypoxic regions (Froehlich et al., 2014). Projections indicate that exposure of all life stages to acidification will increase the most between the present and 2100 (compared to hypoxia and warming; Berger et al., 2021), but the effects of acidified conditions on population distributions and crab catchability during tribal and state fisheries catch seasons in Washington is currently unknown (cf. Froehlich et al., 2017).
These factors highlight a need for closer interaction between water quality and fisheries management research and application to address the complex evolving multi-stressor seascapes facing regionally important species. Differences between northern and southern Olympic Coast acidification mean conditions and seasonality across time suggest an opportunity to use this region as a testbed for understanding how small-scale regional variations in ocean conditions may affect realized future climate impacts to fisheries. Projected economic impacts to Dungeness crab fisheries show substantial differences in northern versus southern Washington fishing ports (Hodgson et al., 2018). Dungeness crab population data collected by fisheries managers could be compared to estimates of acidification-related water quality changes across decades as derived here to assess whether Dungeness crab population distributions and availability to fisheries may be changing in ways that could reflect changes in exposure to increasingly acidified conditions over recent decades and going forward into the future.
Further, US water quality regulations require states to assess pH impairment for coastal water bodies based on change from natural condition (i.e., –0.2 pH change from a pre-industrial reference) or using a “narrative criterion” reflecting the health of biological communities (e.g., Weisberg et al., 2016). These new pH estimates show that in deeper water, where variability is lower, change from a pre-industrial reference state was already discernible at a one standard deviation level during the upwelling season in index year 2010 (Figure 3b,d). However, estimates for 2020 generated in the same way (not shown) indicate that mean conditions may have already exceeded the pH “change from natural condition” standard during two to five months across all four sites. The most acidified deep southern location had the only 2020 July–August monthly averages that did not meet this criterion. By 2030, all upwelling season monthly means may exceed the –0.2 change criterion if oceanographic conditions and water masses remain similar to the 2006–2019 mooring period.
Using this information in tandem with information from fisheries about Dungeness crab population changes could also inform fisheries management approaches and “narrative criterion” assessments. In the context of the regional Dungeness crab fishery, these new results illustrate small-scale variation in how ocean acidification is evolving in a region that relies on healthy fisheries. Collectively, these results point to a need for more coordinated and enhanced monitoring of multiple stressors and species, as well as better integration between water quality and fisheries management to address how changing ocean conditions are affecting important managed resources (e.g., Schindler and Hilborn, 2015).
Support Needed for Resource Managers Facing Accelerating Ocean Change
Over the last 15 years, PMEL ocean carbon scientists have worked with a broad range of research partners and data end users to co-develop decision support information and tools that can facilitate healthy resource and ecosystem management in a rapidly changing coastal ocean. These partnerships have built on a foundation of decades of open-ocean observing highlighted in other articles in this special issue as well as extensive regional partnerships (e.g., Newton et al., 2022). Here, we show that Olympic Coast benthic habitats exceed acidification thresholds relevant to Dungeness crab and their prey earlier and longer than they did in the pre-industrial era. Comparing these new pre-industrial estimates with projected end-of-century conditions highlights the vastly different ocean conditions coastal ecosystems and species will face, with strong declines in pH and Ω, amplification of pH and [H+] seasonal amplitudes, and corresponding attenuation of Ω seasonal amplitudes. Using these new estimates of ocean acidification change from the pre-industrial era to 2100 as a lens for evaluating evolving Dungeness crab exposure to acidification illustrates an approach that tribal, state, and federal water quality and fisheries managers could use to chart a path toward better-integrated resource management in the face of accelerating ocean change. Dynamic regional oceanographic conditions, diverse end users of relevant information, and complementary research assets present opportunities for PMEL to engage in future partnerships to test proposed adaptation, mitigation, and carbon dioxide removal approaches, and to develop resilient and adaptive management approaches for our future coastal oceans and ecosystems.
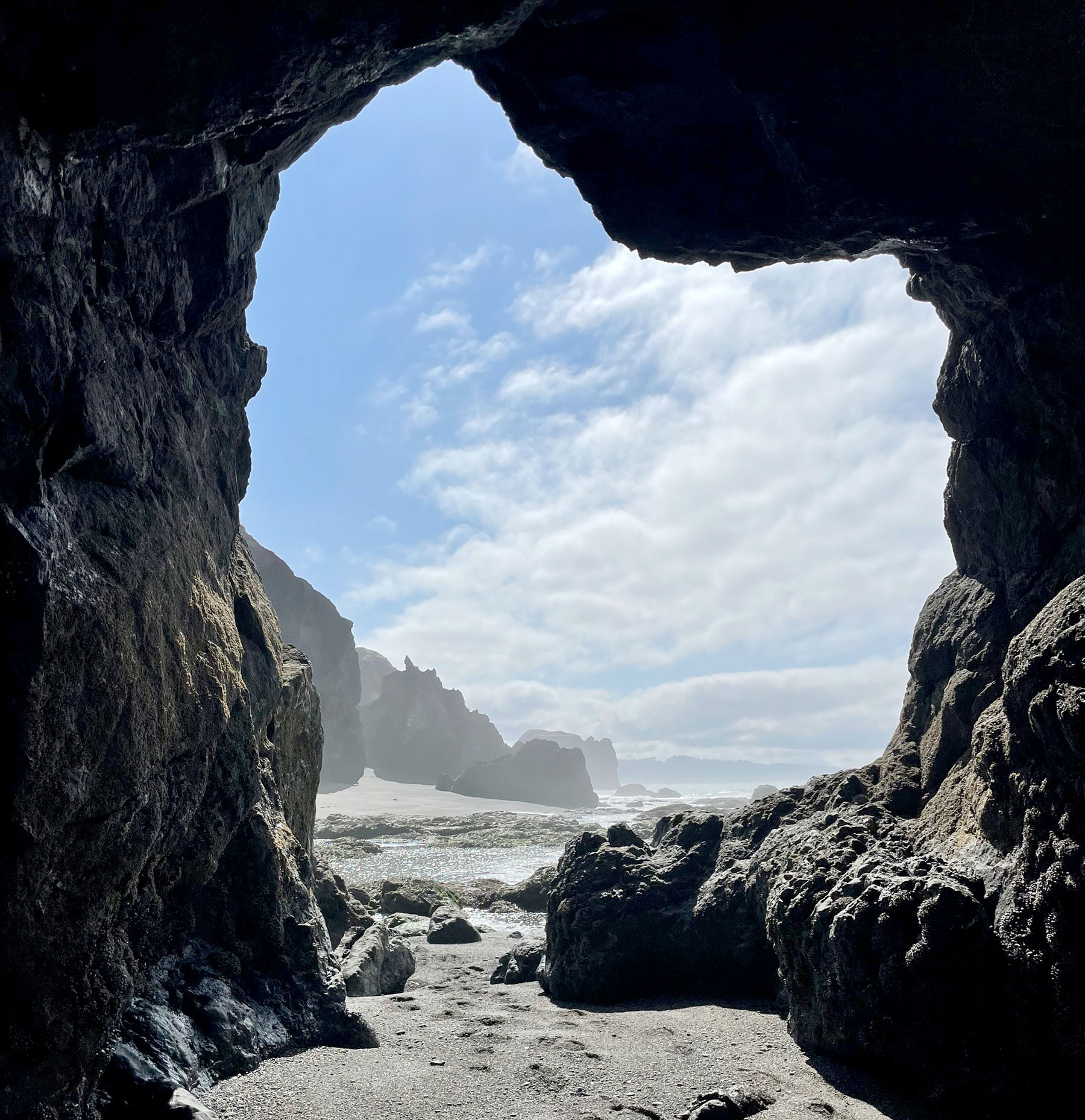
A view from Point Grenville, where the Quinault Indian Nation has lived and harvested marine resources since time immemorial. Photo credit: Katie Wrubel. > High res figure
|
Acknowledgments
Special thanks are due to the dedicated members of the OCNMS field team, especially Kathy Hough, Patrick A’Hearn, and a series of intrepid NOAA Corps officers, for their efforts to both collect and share the sanctuary’s long-term mooring data that made these analyses possible. We are grateful to the NOAA Office of National Marine Sanctuaries (ONMS) for supporting the moored observations; NOAA Ocean Acidification Program (OAP) for supporting the Olympic Coast Regional Vulnerability Assessment co-led by Jan Newton and Melissa Poe; NOAA National Centers for Coastal Ocean Science, Climate Program Office, OAP, ONMS, and Integrated Ocean Observing System for supporting the Northern California Current Multi-Stressor project co-led by Francis Chan and Richard Feely that will continue this work; the NOAA Ocean Acidification Program and NOAA Modeling, Analysis, Predictions, and Projections Program for supporting co-development of decision support tools for fisheries managers; the Schwab Charitable Fund, via the generosity of Wendy and Eric Schmidt, for supporting climate projections in the Cascadia model; and NOAA Pacific Marine Environmental Laboratory for salary support for Simone Alin and Richard Feely. The University of Washington Coastal Modeling Group developed the Cascadia ROMS model used for end-of-century acidification projections. Chris Sabine, Carol Bernthal, Mary Sue Brancato, Liam Antrim, and George Galasso contributed to developing the original PMEL-OCNMS partnership. We thank Adrienne Sutton and two anonymous reviewers for constructive comments that improved the manuscript. This work constitutes PMEL and the University of Washington Cooperative Institute for Climate, Ocean, and Ecosystem Studies contribution numbers 5944 and 2023-1259, respectively.
Disclaimer
The scientific results and conclusions, as well as any views or opinions expressed herein, are those of the author(s) and do not necessarily reflect those of NOAA Oceanic and Atmospheric Research or the Department of Commerce.