INTRODUCTION
NOAA relies on an integrated suite of observations and nationwide observational systems. In 2015, the NOAA Pacific Marine Environmental Laboratory (PMEL) initiated a new program to facilitate Arctic-focused observing solutions for NOAA: Innovative Technology for Arctic Exploration (ITAE). PMEL housed the science and engineering expertise needed to facilitate the emerging push to develop observational infrastructure for the US Arctic. The first Arctic Vision and Strategy report was released in 2011 (NOAA, 2011). It was followed by the 2013 release of the National Strategy for the Arctic Region (Obama, 2013). At the time, there was widespread interest in environmental, economic, and national security issues in the US Arctic. The formation of a collaborative program to facilitate rapid technology development to aid in Arctic research ensured that NOAA could gather the data needed to inform responsible Arctic resource management (Cross et al., 2015).
Of the large portfolio of projects, the “big three”—the saildrone uncrewed surface vehicle, the Oculus glider, and the Alamo float—defined the first of the autonomous and uncrewed system projects in the ITAE portfolio (Figure 1). Simultaneously, the development of small-scale sensors (though not to be confused with small-scale projects) tackled long-standing scientific gaps to support operational systems at NOAA (e.g., weather, ecosystem health, ecosystem modeling, and fisheries).
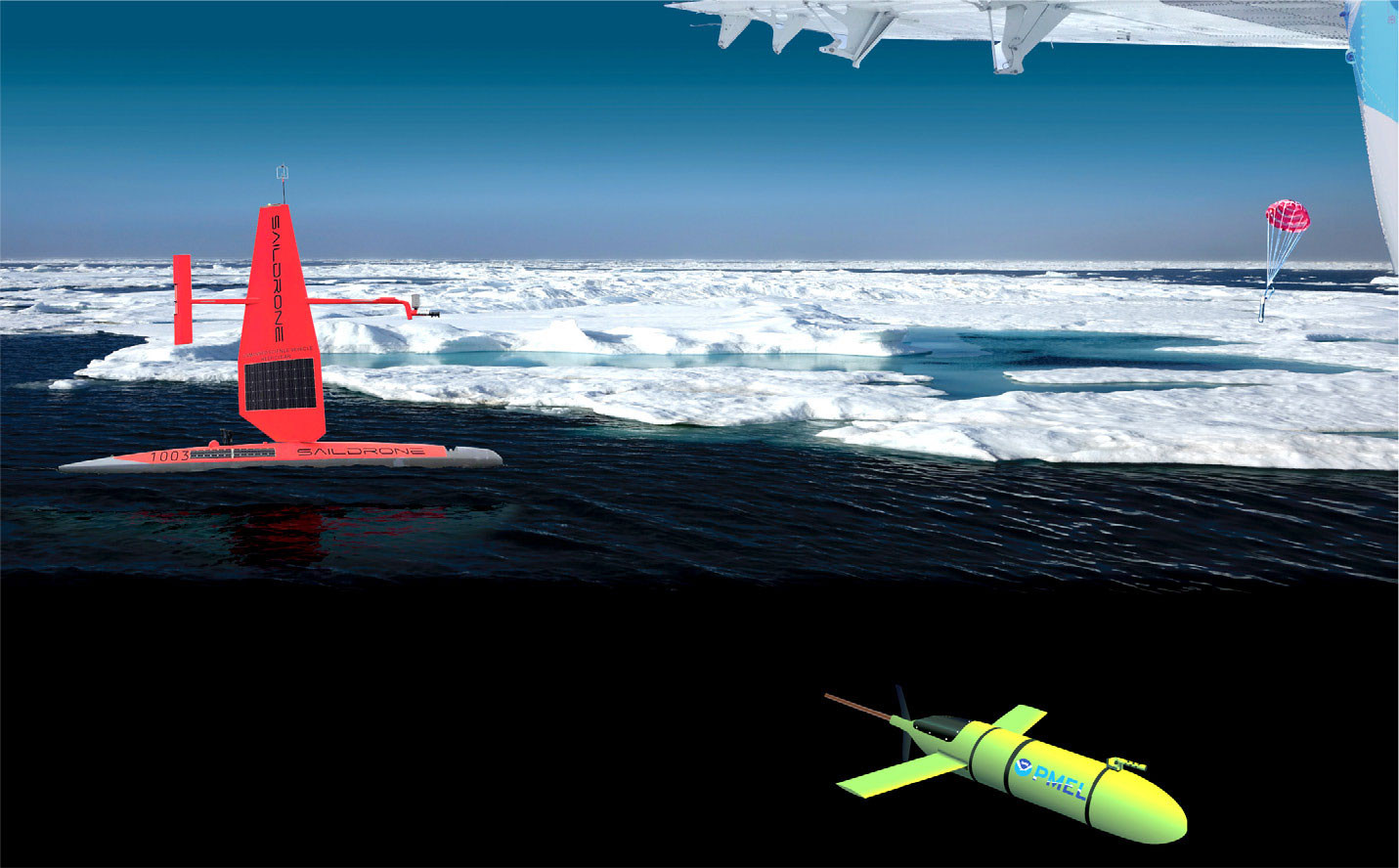
FIGURE 1. Arctic conceptualization of three technologies that have been successfully deployed and operationalized for use pole to pole. The uncrewed surface vehicle saildrone, from Saildrone Inc., is shown at left, in orange/red, the Oculus shallow-water glider appears bottom right, neon yellow, and the blue ice-hardened Alamo profiling float lands upper right with its red parachute. The latter was developed with MRV Systems in partnership with Woods Hole Oceanographic Institution. > High res figure
|
As part of this PMEL 50th anniversary issue, we provide a retrospective of the ITAE program, lessons learned, and what is next.
HOW ITAE WORKS
The ITAE program supports NOAA’s research and development enterprise by accelerating and facilitating the transition of Arctic-focused observing assets for wider use through public-private partnerships, platform and small-scale sensor design, research to operations (R2O), research to commercialization (R2C), and research to application (R2X) of science-driven technologies (Figure 2). The program values high-risk, high-reward projects. ITAE leverages the expertise of personnel from NOAA Cooperative Institutes, PMEL programs, NOAA line offices, other government agencies, academia, and industry with diverse engineering skills, infrastructure, and technological assets.
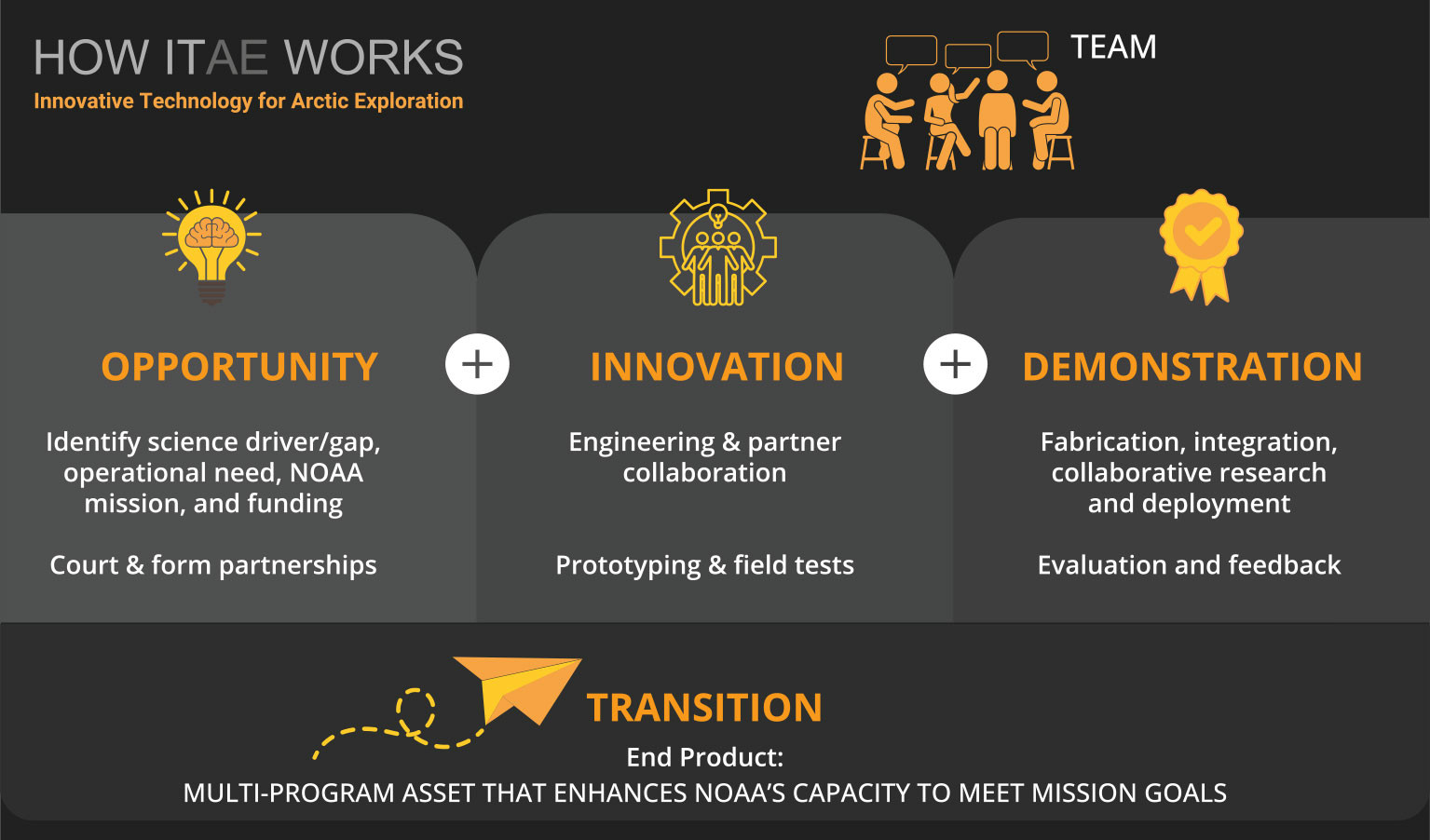
FIGURE 2. Diagram depicting how the Innovative Technology for Arctic Exploration (ITAE) program works across the four stages of project development: opportunity, innovation, demonstration, and transition. Such a collaborative is composed of a four-person core team at the NOAA Pacific Marine Environmental Laboratory, Seattle, Washington, USA. > High res figure
|
To maintain a diverse portfolio of research and development, ITAE has supported each NOAA line office in varying capacities. From testing products such as ocean exploration mapping with the National Ocean Service to integrating measurements on the Global Telecommunications System with the National Weather Service; validating satellite data with the National Environmental Satellite, Data, and Information Service; supporting emerging fisheries research applications with Fisheries; and conducting foundational research with the Office of Research, the breadth of both primary and ancillary projects have laid the foundation for many of the current advancements in observing assets (e.g., saildrone, plankton imaging, primary production index).
Because of the collaborative nature of the technological developments, the ITAE program is able to leverage twice its operating budget. At one point, the program connected over 45 partner programs through the big three, and at times, more than 100 people were collaborating across the various projects. These large numbers were primarily driven by the 2016–2018 saildrone field testing and expansion of the core sensor suite as well as small test-project requests for potential users at NOAA (e.g., National Ocean Service single-beam mapping).
A good partner, and reflection and growth in times of failure are integral part of a successful ITAE project. Good partners establish and align vision and goals. From transparency to determination, formation of a team of experts with early-stage collaboration generates a synchronized working team and avoids process breakdown. The formation of Cooperative Research and Development Agreements (CRADAs) are also an essential component of engineering co-development for platforms and sensors. CRADAs should be considered alongside early-stage writing of transition plans within NOAA.
Collaborations add significant value to projects, especially when field testing and operationalizing ocean observation assets in the US Arctic. Regionally, research in the US Arctic is ice-driven, limiting ocean field measurements to ice-free months (April to October). Because the US Arctic is remote and covers a vast region, the research network there is highly utilized. Established communication pathways and feedback loops strengthen collaborations and assure mission-focused decision-making.
The ITAE core team is composed of three principal investigators and a program coordinator. Each project has a lead scientist, a lead engineer, and a lead representing the core ITAE program as a project facilitator. ITAE aims to provide a diverse portfolio to support large and small projects that meet program criteria.
Several projects have also been conducted in collaboration with private sector companies that can quickly harness capital and build assets, shouldering a significant amount of development and management risk. Meanwhile, the public sector scientific community provides guidance and shares expertise to ensure that any new assets or protocols meet data quality and access standards and target mission goals. While public-private partnerships have been centered primarily around uncrewed platforms (large-scale projects), it is the small-scale projects (e.g., sensor design) that may have the largest impact on research in the US Arctic.
TRACKING DEVELOPMENT
ITAE is uniquely positioned to serve as an integrated transition enterprise inspired by gaps in US Arctic science as well as the Arctic as a test region while connecting to the current NOAA mission and vision documents. This includes (but is not limited to) the NOAA Research and Development Vision Areas: 2020–2026 (NOAA Research Council, 2020) and NOAA’s Arctic Vision and Strategy (NOAA, 2011). Project maturation is tracked through NOAA readiness levels (RLs; NOAA Office of the Chief Administrative Officer, 2019). These are similar to technology readiness levels developed by NASA (Mankins, 1995) and embody the same concept for quantifying the maturity of research. However, NOAA RLs further integrate components to support NOAA’s unique research and development enterprise.
RLs are used to characterize the status of research and development relative to its readiness to be used operationally or in other societal applications. Nine RLs span four categories: research, development, demonstration, and deployment. RLs are not linear, nor is advancement always linear. Projects may enter the development pipeline at any of the nine stages. Projects that fail to mature will be terminated, and in some cases transferred back to an external partner.
ITAE is also designed to bridge the “Valley of Death,” a metaphor commonly used to describe a lack of resources to bring good ideas to fruition in both public and private sectors (e.g., Finkbeiner, 2010; Barron and Amorós, 2020). Sustained funding provided to ITAE and tracking of each innovation through the RL steps reduces failures that might otherwise occur. More recently, ITAE works closely with the Office of Research, Transition, and Application (established in 2022), which provides transition planning and readiness level guidance to accelerate and facilitate transitions of research and development within NOAA.
Each matured project has a transition plan, which includes general information about the project and a proposed process for how it can most efficiently be integrated into operations or other applications. This plan constitutes an agreement between identified researchers (the “team”) and includes potential recipients, organizations, or other users of the product. Early (and often) coordination during a project ensures researchers, developers, and operators identify both the potential for success and possible limitations of both the new technologies/ideas and the existing operational framework. This plan allows NOAA to ensure successes in its own agency-wide portfolio and that federal taxpayer funds provide benefits to society.
One strategy ITAE employs to reduce failures is to focus on science-driven innovations in consultation with an end user to identify a critical research gap that aligns with NOAA and PMEL missions and to determine how the product can best be utilized once transitioned. This science-driven partnership approach helps to eliminate stagnant projects and promotes common goals and values. This also allows a focus on maritime technology to understand and predict changes in climate, weather, the ocean, and coasts, thereby contributing to economic growth, ocean health, and ocean management in the US Arctic.
The ITAE Portfolio
The New Blue Economy
NOAA’s research and development projects provide critical scientific knowledge and tools that support sustainable economic growth and environmental stewardship within the new blue economy. Effective long-term management of the blue economy requires regular and accurate observations of essential climate and ocean variables (Task Team for an Integrated Framework for Sustained Ocean Observing, 2012); however, cost and regional and seasonal logistical challenges can be barriers to collecting observations in the Arctic. Climate change’s rapid and accelerating pace is driving increased demand for information about the ocean and our coasts. Increasing numbers of autonomous systems are rapidly transforming our global understanding of coastal and marine ecosystems. These technologies can collect large amounts of data for use in mitigating risks from hazardous weather and other environmental phenomena, foster the sustainable use and stewardship of ocean and coastal resources, and provide a greater understanding of Earth systems. ITAE supports efforts that gather ocean data and information to address societal challenges and contribute to their solutions.
Public-private partnerships provide opportunities to blend expertise, reduce risk, and enhance economic growth. Project teams can quickly build, deploy, and apply high-quality scientific tools that both serve the public good and meet private sector needs. The public-private partnership with Saildrone Inc. is a gold standard example of this type of effort (Meinig et al., 2019; Zhang et al., 2023, in this issue). Saildrone pushed technical and endurance boundaries in order to collect critical ocean data in locations such as the Arctic that have traditionally been out of reach or prohibitively expensive places to work. ITAE provided the funding, coordination, and expertise to develop the saildrone core sensor suite. The program also provided an initial testbed for fish surveys, charting and ocean mapping capabilities, collection of carbon measurements, and the integration of measurements into the Global Telecommunications System. The public-private partnership with Saildrone Inc. has forged a new path of data-as-a-service for NOAA (Meinig et al., 2019). Data collected using saildrone technology has also provided groundbreaking insights into inform conservation management decisions in Alaska (De Robertis et al., 2019; Kuhn et al., 2020) and has improved nautical charts.
Gliders are increasingly practical tools for supporting ecosystem research and enhancing observing capacity. Development of the Oculus glider began in 2015 to support US Arctic research with a workhorse uncrewed system that did not rely on ship deployments and could operate in shallow coastal waters. These gliders provide real-time, high-resolution data that support NOAA’s only biophysical mooring array in US waters, enhance understanding of how dynamic features such as ice and weather events affect marine ecosystems, improve predictive models, and document natural variability, contributing to foundational research that supports fisheries resource management decisions in regions such as Alaska. Now, eight years later, following successes (four gliders built and operational) and failures (the intellectual property and building of the glider will no longer be supported by a commercial company), the integration of the glider into NOAA US Arctic operations is ongoing.
Ocean Health and Management
Although most autonomous systems collect some subset of essential ocean variables, sensors can be added to platforms such as saildrones and gliders to target specific parameters of interest (e.g., Meinig et al., 2019). However, ITAE investments in small-scale sensor refinement (in size and power draw) and data-processing methods may have the most impact on ocean health management decisions.
Products from ITAE projects provide important contributions to annual Ecosystem Status Reports (ESRs). ESRs convey information on the status and health of Alaska marine ecosystems to the North Pacific Fishery Management Council and other stakeholders, thus providing context for ecosystem-based fisheries management in Alaska. Two examples of ITAE innovations contributing to ESRs are measurements of primary productivity and observations of zooplankton distributions.
Primary production is an essential ocean variable for understanding marine ecosystem functioning in the Bering Sea as shifts in sea-ice cover, seawater temperatures, and nutrient availability occur. To address the need for data on primary production, NOAA scientists partnered with colleagues in academia to create a multi-method determination of primary production in the southeastern Bering Sea. The profiling crawler, or Prawler, was a key technology that enabled this calculation (see Stalin et al., 2023, in this issue). Now sold commercially, the Prawler harnesses wave energy to ratchet a sensor package along the mooring line with shore-based command and control. The Prawler profiles the water column multiple times daily, providing real-time data on ocean temperature, salinity, oxygen, and chlorophyll. These and other parameters are used to provide metrics on gross primary production, net primary production, and net community production for the Bering Sea ESR (Cynar et al., 2022; Nielsen et al., 2023). Prawler data are integrated into NOAA’s observing system and are used by stakeholders and managers.
Zooplankton abundance and species distribution help explain fisheries recruitment. Traditional sampling has utilized oblique net tows, but because it often takes six or more months to fully process resulting zooplankton samples, complete species composition and abundance data are not available to managers. To address this limitation, an onboard, rough count of zooplankton net samples, called the Rapid Zooplankton Assessment (RZA) has been developed. The RZA count is used as an ecosystem indicator by the North Pacific Fisheries Management Council. However, critical knowledge gaps still exist that cannot be addressed through net sampling and the rapid assessment process. The use of imaging systems could replace the RZA by providing more spatially resolved information that is indicative of system productivity. In collaboration with ITAE, zooplankton ecologists are developing an imaging machine learning process for the Bering Sea, and image data will be used to assess the state of the ecosystem and help influence fisheries management decisions concerning total system productivity and allowable catch. This information would enhance the mission of NOAA Fisheries by providing more timely and accurate information to fisheries managers and stakeholders.
In addition to the ESR contributions, other ITAE efforts support fishery management decision-making, including measurements of northern fur seal prey captures. A large proportion of the northern fur seal (NFS) population returns to the Pribilof Islands in summer for reproduction and pup provisioning. The US government has conducted counts of NFS pups since 1912. The population of NFSs has been in decline, even after the hunt was curtailed. Female NFSs undertake foraging trips of 3–10 days in search of walleye pollock, their primary prey. Information was lacking on directly observed prey captures to know whether foraging trips provide enough energy (i.e., prey captures) for moms to nourish their pups to ensure survival on their own during their first winters. In collaboration with ITAE, fish distributions were more clearly established by using saildrones equipped with acoustic fish finders (Mordy et al., 2017), as well as specialized cameras and dive trackers mounted on the animals to determine the fraction of successful foraging dives (Kuhn et al., 2022). This information is helping to validate bioenergetics models, and the results are being provided to fishery managers (McHuron et al., 2020).
GROWTH IN FAILURE
Development and growth nurture risk and temporary setbacks. And failures are inherent in ITAE’s high-risk endeavors. Some failures constitute temporary setbacks along the development-to-transition pipeline but result in overall improvement in design and function.
For example, the Oculus glider uses a high-pressure pump that enables rapid changes in buoyancy for operation in shallow water. In 2017, the glider was deployed for 20 days and completed 1,020 profiles but was then lost because of a leaky valve in the buoyancy engine. This necessitated re-engineering the internal system of the glider, including redesign of the engine, installation of an oil-gauge for monitoring leaks, and a temporary reduction in RL. This failure was overcome, and the Oculus progressed toward transition.
Similarly, the saildrone underwent numerous design modifications to improve efficiencies and adjust for failures during prototype field tests. The team set an ambitious project goal of instrumenting, testing, and deploying a prototype in the US Arctic within 18 months and successfully completed the first field mission in 2015 (Meinig et al., 2019). This led to adjustments to the keel and hull design of the uncrewed surface vehicle, adjustments to battery capacity, additional sensor calibration, and improvement in team communications strategies.
Some projects along the development pathway will fail to meet program requirements and NOAA mission needs—and may be removed from the portfolio, transferred to an external partner, or terminated. For example, ITAE developed a prototype air-deployable ice tracker, but it was found to be unworkable, and the project was terminated at a RL 4 (e.g., successful evaluation of system, subsystem, process, product, service, or tool in a laboratory or other experimental environment—an intermediate phase of development). While this project was innovative, it was not a good fit for ITAE.
Similarly, during field tests of a prototype alkalinity sensor, the sensor was not functioning and developing as hoped and it was not possible to meet mission needs. This effort was concluded at RL 5 (successful evaluation of the system in a relevant environment through testing and prototyping; this can be considered the final stage of development before the demonstration begins), although the research need still exists. The ability to measure total alkalinity is a crucial requirement for understanding the variability of calcium carbonate saturation states and ocean acidification in the world ocean, as well as the impact of ocean acidification on some shell-forming organisms. Testing this sensor in the Arctic had the potential to ensure use beyond the easy-access and warm-water environments for which ocean technologies are typically designed.
The National Oceanography Centre in the United Kingdom provided a prototype in 2015 of a Lab-on-a-Chip nitrate sensor that ITAE field tested on a mooring in the Chukchi Sea. While the sensor functioned well, it was evident that the ITAE-driven miniaturization of this sensor for intended platforms (e.g., saildrone, glider) was not ready to be incorporated, and it was removed from the portfolio. However, the associated assessment of data and collaboration gave the PMEL nutrient laboratory sufficient confidence in the sensor to purchase several units (once the product was commercialized in 2023) for deployment on moorings in the Arctic.
Another promising project for examining nutrient dynamics in the polar regions of the ocean is a moored, in situ nitrification incubation system for measuring how rapidly nutrient levels are reset in winter to support primary production, and ultimately fisheries, the following spring. Although the moored system was verified in Hood Canal, Washington (locally), and deployed several times in the US Arctic, it has been met with difficulties and extensive delays in sample processing.
Conclusion
ITAE is an example of a successful high-risk, high-reward program that operates using a core set of values aligned with NOAA missions to push the boundaries of ocean observing. Where other established programs look for more mature projects, ITAE can enter at the earliest stages and support the vision through various development hurdles while also providing collaborative expertise and connections with end users.
The future of the program will rely heavily on strong collaboration and integration with aligned NOAA programs as well as potentially feeding from incubator programs both locally and federally in a formal capacity. The next biggest thing may not be an uncrewed system, but perhaps a smaller innovation as the program moves forward, leveraging partner assets and developing an integrated pipeline.
The new challenges climate scientists and engineers are facing will help reimagine how we support innovation. Reliable measurements require expertise and diligence. Formalizing partnerships, identifying use cases, and enhancing transparency are keys to success in science-driven engineering development and partnerships. Solutions should always be a guiding principle in science-driven technology and sensor development. Through maximizing existing and establishing new partnerships, NOAA can build strong coalitions to address the greatest needs in ocean research and serve US interests over time. Looking ahead, ITAE stands ready to support a sustainable and equitable ocean and coastal economy that optimizes advances in science and technology by creating value-added, data-driven solutions to pressing societal needs.
Acknowledgments
We thank Chris Meinig and Jessica Cross who were previously PIs with ITAE. We thank our many partners and teams, past and present. We also thank the anonymous reviewers and guest editor for their careful reading, and for helpful, constructive suggestions that strengthened this paper. This program is a multi-institutional collaborative effort across internal NOAA line offices, NOAA Cooperative Institutes, and external collaborators. ITAE is funded by NOAA and partially funded by the Cooperative Institute for Climate, Ocean, and Ecosystem Studies (CICOES) under NOAA Cooperative Agreement NA20OAR4320271. This is contribution 2023–1262 to CICOES and 5493 to PMEL. The scientific results and conclusions, as well as any views or opinions expressed herein, are those of the authors and do not necessarily reflect those of OAR or the Department of Commerce.