Introduction
The articles in this special issue of Oceanography provide an extensive overview of the Gulf of Mexico science community’s accomplishments. Much of the work discussed in this article was funded by the Gulf of Mexico Research Initiative (GoMRI), created in response to the Deepwater Horizon (DWH) disaster that began on April 20, 2010. Zimmermann et al. (2021, in this issue) summarize the genesis and operation of GoMRI, and McKinney et al. (2021, in this issue) describe the complexity of the Gulf of Mexico ecosystem. Here, we provide a high-level overview of the major outcomes of the studies conducted by GoMRI researchers whose work advanced existing paradigms in oil spill science and extended aspects of findings from previous studies. These outcomes, in combination with the other GoMRI synthesis products (https://research.gulfresearchinitiative.org/research-awards/gomri-synthesis-projects/) represent an important and simultaneous accomplishment in advancing the overall breadth of general scientific knowledge across the physical, chemical, geological, and biological oceanographic research fields, as well as in related technology, socioeconomics, human health, and oil spill response measures.
With over 4,500 scientists and experts involved with GoMRI, the program funded nearly 300 projects that resulted in more than 1,700 peer-reviewed articles. These projects helped educate and train some 1,400 MS and PhD students and 1,050 undergraduates. While the majority of researchers were located in the Gulf of Mexico region, the program included participation from researchers in 43 states and 17 countries. This colossal effort not only fostered collaboration among researchers located at institutions within the Gulf of Mexico region but also established national and international research connections that will facilitate future research endeavors.
GOMRI-funded research was interactive, especially in the first few years, with research and related activities funded by BP, along with the National Science Foundation’s Rapid Response Research (RAPID) grants program, the Natural Resources Damage Assessment, National Oceanic and Atmospheric Administration (NOAA) Sea Grant college programs, and several other federal, state, and nongovernmental organizations that contributed to advances in oil spill science.
Physical Processes
Physical transport processes such as the circulation and mixing of waters largely determine the spatial distribution of materials in the ocean. These processes establish the physical environment within which biogeochemical and other processes transform materials that include naturally occurring nutrients and human-mobilized contaminants. An understanding of these physical driving forces and their influence on biological, geological, and chemical processes was significantly advanced through improvements made by GoMRI researchers to models representing the transport of materials.
Boufadel et al. (2021, in this issue) review the physical processes that are responsible for distributing oil, dispersant, and related decomposition products. These processes operate on a very wide range of scales, from the microscale of droplets and bubbles; to small-scale turbulence in buoyant plumes and the near-surface “mixed” layer; to the submesoscale of fronts, convergent and divergent flows, and small non-geostrophic eddies; to the mesoscale of larger quasi-geostrophic eddies; to the large-scale circulation of the Gulf of Mexico and its interaction with the Atlantic Ocean and the Caribbean Sea. The circulation and mixing processes near the Gulf’s coasts are strongly affected by wind- and river-
induced currents, and further modified by its complex seafloor topography. Gulf of Mexico physical processes are further characterized by strong linkages between coastal/shelf and deeper offshore waters that dictate connectivity to the basin’s interior. This physical connectivity influences the transport of materials among different coastal areas within the Gulf and also to adjacent waters. GoMRI scientists have made major advances in observing, understanding, and modeling all aspects of the physical environment. These advances include development of new tools and methodologies for collecting, analyzing, and synthesizing data, and techniques for capturing coastal to offshore deep-sea linkages and transport.
Advances, Discoveries, and Surprises
Among many other advances, GoMRI contributed to the understanding of how pressure-induced processes affect gas-saturated oil. Refinements of transport modeling tools have materially improved abilities to predict Gulf of Mexico circulation and oil transport, ecosystem connectivity, and ecological impacts. The application of detailed computational fluid dynamics (CFD) modeling has resulted in a transformational shift in the ability to model complex multi-phase (oil, gas, and water) plumes using numerous methods over a wide variety of environmental conditions. CFD models demonstrated the unexpected importance of Earth’s rotation on the near-field plume dynamics, affecting both entrainment rates and detrainment levels.
GoMRI-funded scientists developed new biodegradable drifters and used them to measure and constrain models of small and submesoscale ocean surface currents in unprecedented detail. This advancement allowed researchers to explore implications for oil transport at a level of complexity that was novel to physical oceanography. Field observations and models of near-surface, submesoscale processes, such as Langmuir circulation, have shown the previously under-appreciated importance of vertical rise velocity in controlling dispersion and surface distribution of buoyant materials such as oil.
A new-found appreciation was developed for the important role that river-induced fronts play in longshore and cross-shore transport, acting both as barriers to material transport and as convergence zones that guide transport along frontal lines. Researchers now have explicit data on advection of pollutants along frontal pathways that link coastal areas, and on how both mesoscale and submesoscale circulation features influence the open ocean. These new data greatly improved scientists’ understanding of transport because they were directly examined over a long period on a variety of scales.
For the larger (mesoscale and beyond) spatial scales, predictive improvements have been made through use of high-resolution regional modeling (improving from 4 km to 1 km resolution). This advance incorporated river-estuarine coupling, the ability to resolve both the surface and the bottom Ekman layers, explicit consideration of Stokes drift, and the widespread use and assimilation of operational meteorological and reanalysis products.
Lessons for the Future
The use of river diversions was ineffective in achieving the objective of pushing DWH oil away from coastal wetlands and resulted in unanticipated deleterious ecological impacts. The potential consequences of diversions need to be more carefully considered before they are implemented.
We also learned that accurate prediction of oil transport at the surface can only be achieved by using the best available high-resolution models combined with real-time assimilation of comparable-resolution data and paying careful attention to the effects of winds, waves, Stokes Drift, and submesoscale motions in the near-surface layers. The deployment of large numbers of floats in real time was shown to be feasible and efficient, and thus can provide an excellent source of additional information on near-surface circulation.
Scientists quickly learned that significant quantities of contaminants from a deep spill can be carried laterally over large distances in subsurface plumes as both dissolved and particulate material. These contaminants can then be deposited on the continental slope within a restricted depth range and consequently impact organisms living there.
Future Research Needs/Gaps
While numerous advances have been made, predicting the distribution of spill products remains challenging. We consider the following areas of further research particularly important:
- Improving methods for both observing and modeling velocity profiles in the uppermost meter of water, where changes may be both large and transient
- Improving the time-varying estimation of initial droplet size distribution as live oil rises from the buoyant plume into the far-field water column
- Quantifying the impact of turbulent thermal wind effects on frontal turbulent intensities and the evolution of submesoscale fronts
- Improving algorithms to achieve a multiscale modeling approach for simulating wetlands, estuaries, river-estuary coupling, riverine forcing, and shelf exchanges, and their linkages with open ocean transport
- Improving understanding of the role of bottom-boundary dynamics, where model parameterizations remain underdeveloped and data scarce
- Collecting additional field data from shelf breaks at critical locations to constrain exchanges between estuarine, coastal, and open ocean regions
- Integrating all model improvements into an operational Earth system modeling framework (ocean-atmosphere-waves-biogeochemistry)
To sustain future growth and amplify benefits, we recommend developing and sustaining regional forecast systems that are based on a suite of appropriately scaled and nested ocean circulation, atmospheric, and wave models. This system should be capable of strategically focusing on the key components of the land-ocean continuum and supported by well-designed observing arrays targeting process understanding, data assimilation, and model validation.
Chemical and Biogeochemical Processes
Petroleum is an extremely complex mixture of many thousands or more of gaseous, liquid, and some solid constituents. The chemicals in oil are modified while being transported to and through different environments. These changes occur due to natural processes (e.g., photo-oxidation and other forms of weathering), biological transformations (e.g., microbial degradation and decomposition), and via response measures (e.g., in situ burning). The oil constituents and their reaction products are transported by processes described above (see section on Physical Processes). Adding to the significance of the DWH oil spill is the sheer quantity of petroleum released from the Macondo petroleum reservoir in an uncontrolled way, making it the largest incident of this kind in history (Rullkötter and Farrington, 2021, in this issue.) The scale of the DWH disaster, the time over which it occurred, the geographical extent of the spread of oil, the weathering and biodegradation, and the variety of ecosystems affected presented challenges to a comprehensive understanding of the fates of the materials released to the environment.
Resuspension, horizontal transport, and redeposition moved sediment and seawater contaminated with weathered and biodegraded Macondo oil away from continental shelf areas to continental slope and abyssal areas, as well as onshore. Continental slope sediments were also contaminated with deposition from overlying waters or by impingement of the subsurface plume in some areas.
Understanding these processes was further complicated by the unprecedented volumes of dispersant (Corexit 9500, 9527) that were sprayed from planes and boats onto surface slicks as well as applied directly at the wellhead through subsea dispersant injection (SSDI) at about 1,500 m water depth.
The interrelationship between gas bubbles, oil droplets of various sizes, and early dissolution of petroleum components in the water column immediately adjacent to the damaged wellhead, with and without dispersants, was a major barrier for trying to understand the spill. Although both vertical and horizontal plumes were anticipated, their scales and extents were not. The processes determining these interrelationships were the subject of modeling and laboratory simulations. The expected dissolution of chemicals in subsurface waters was confirmed by field sampling and analyses at varying distances and depths away from the well site (Farrington et al., 2021, and Quigg et al., 2021, both in this issue).
Advances, Discoveries, and Surprises
Significant progress in assessing the fate of spilled petroleum components was made, and several important lessons were learned and discoveries made that could pertain to future spills of various sizes. Over this past decade, scientists have followed the distribution and fate of Macondo oil in the water column and sediment. Scientific studies have described the role of photo-oxidation of petroleum compounds at the sea-air interface and the roles particulates and microbes play in the fates of hydrocarbons. These studies included various documented processes, such as marine oil snow (MOS) formation and marine oil snow sedimentation and flocculent accumulation (MOSSFA), that expanded our understanding of hydrocarbon deposition and accumulation in sediments, fates on beaches and in marshes, and bioaccumulation.
Depending on sunlight irradiance and weather conditions, photo-oxidation was a significant process at the sea surface in the breakdown and fate of spilled oil. Despite earlier research in the 1970s and 1980s noting the sensitivity of petroleum to photo-oxidation, the related effects were downplayed and grossly underestimated in terms of mass balance significance over more than a decade (Transportation Research Board and National Research Council, 2003). As a result of recent advancements in chemical analysis, especially Fourier-transform ion cyclotron resonance mass spectrometry (FT-ICR-MS), this underestimation has been corrected so that we now have a better understanding of the large numbers and types of breakdown products (Rullkötter and Farrington, 2021, and Farrington et al., 2021, both in this issue). Photo-oxidation products were found to be significant components in weathered oil on contaminated beaches and in marshes affected by the oil spill. Resultant contamination of the surface sediments was very patchy, but measurable, in several places and lasted in some areas for three years or more. Contamination of the subsurface sediments persists and is expected to be long lasting.
The microbial community was also a significant contributor to the fate of Macondo oil. Hydrocarbon-degrading microbes efficiently remove hydrocarbons from the water column, with some degradation products being directly incorporated into marine food webs. Surprisingly, microbially mediated MOS was documented as an important factor in the biogeochemistry and fate of oil spilled to the marine environment in continental margin and deep ocean ecosystems. Similarly, the biogeochemical processes of MOSSFA and its role in transport was better elucidated and exceeded marine snow-associated oil transport estimates of the past (Farrington et al., 2021, in this issue). It was concluded that MOS and MOSSFA processes and associated biodegradation were also affected by interactions of Macondo oil with dispersants.
The vulnerability of coastal ecosystems to oiling has been the subject of decades of research. The oiling of beaches is problematic from human health, commercial tourism, recreation, and aesthetic perspectives (Sandifer et al., 2021, in this issue). Oiled beaches in the Gulf coast region were subjected to extensive cleaning activities during the DWH spill response. Despite these efforts, small residual oil particles in sand known as sand-oil aggregates and small tar patties remained for years and can still be found. In part, this was due to the action of storms uncovering buried oiled sand and moving oil-sand accumulations from shallow-water nearshore regions onshore. The degree of resuspension following storms and hurricanes needs to be accounted for in future planning for response, mitigation, and remediation activities.
Despite the intense response efforts to protect marshes, one of the most challenging ecosystems for removal of oil, significantly oiled areas persisted (Rabalais and Turner, 2016; Halanych et al., 2021, in this issue). One GoMRI project focused on Barataria Bay, Louisiana, and documented that initial oiling of marsh edges was soon spread to greater areas within the marsh by tides and storms and then tidal flows. Oil residue concentrations decreased markedly during the first few years but were still approximately a factor of 10 higher than background concentrations when last sampled eight years post spill. Weathered oil also remains buried in the marsh sediments along the shoreline (Halanych et al., 2021, in this issue).
Corexit 9500 dioctyl sodium sulfosuccinate surfactant, or DOSS, components were found at a considerable distance from the DWH well in the deepwater plume, with oil residues in sediments and also on some deep-sea coral communities. Future research should consider that reaction products resulting from photo-oxidation of surface slicks and films may interfere with efficacy of dispersant applications. Soluble chemicals from in situ burning were found beneath the slick and as residue within experimental simulations (Rullkötter and Farrington, 2021, in this issue).
Lessons for the Future
Several important lessons were learned regarding the conduct of and advances in oil spill chemistry research. Newer analytical methods, for example, GC×GC-MS and FT-ICR-MS as discussed in Rullkötter and Farrington (2021, in this issue), present significant opportunities to advance knowledge of the composition of crude and fuel oils and their weathering and biodegradation products. Concerning chemical techniques, scientists should be mindful of the lack of spatial uniformity in the molecular weight range and individual compounds analyzed in field samples and in mesocosm/microcosm and laboratory experiments. Need for this awareness also applies to the variability in chemical techniques, which hampers cross-comparison of results among different studies (i.e., variability between FT-ICR-MS and gas chromatography-mass spectrometry [GC-MS] used to detect and quantify hydrocarbon compounds; Rullkötter and Farrington, 2021, in this issue). The key new findings noted above, especially regarding photo-oxidation, MOS formation, and MOSSFA, need to be incorporated in a practicable manner into oil spill response, mitigation measures, and assessment models, with appropriate attention to the various types of ecosystems and climates where spills may occur.
The development of extensive data archives has proven helpful for follow-on research by the scientific community at large in unraveling details of the biogeochemical cycles of Macondo oil chemicals. GoMRI data will be broadly used by others for years to come (Zimmermann et al., 2021, in this issue). Given the advances noted above, it is important to employ this information for future spills in order to develop useful and accurate ways to detect, quantify, and budget the fate of spilled oil over time. Bulk oil budget estimates not based on compound-specific analysis are thus problematic. Moreover, any one compound may have successively different fates—a simple example is initial photo-oxidation at the sea surface followed by partial microbial degradation, incorporation into marine snow, and deposition on the seafloor (Farrington et al., 2021, in this issue).
Proper measurements are needed in the field to document the chemical composition and environmental distribution of in situ burning products. It is equally critical to follow the transport pathways of residues from burning as they enter the water column and are eventually deposited as sediment, even in deeper waters (Rullkötter and Farrington, 2021, in this issue).
Concerning the application of surface and subsea injection of dispersants, patent laws and confidentiality regulations initially made it difficult to obtain precise data quickly and reliably on the actual chemical formulation of dispersants used. This lack of information hampered research on the detection, decomposition, fate, and effects of oil and dispersants in parallel laboratory and mesocosm studies.
Future Research Needs/Gaps
Future efforts should seek out new ways to further interpret and use the complex data from FT-ICR-MS analyses of high-molecular-weight constituents of crude oils, fuel oils, and their photo-oxidation products. Beyond the large number of very useful elemental composition data provided by FT-ICR-MS, there is the need for more exact chemical structures, for example, by combining FT-ICR-MS with other analytical techniques. This knowledge gap hampers the understanding of reaction pathways, reaction rates, and the potential for effects of reaction products on organisms and for human health concerns. Additional areas of research include the following list.
- Field measurements accompanying in situ burning of spilled oil are scarce but are urgently needed to document the chemical composition and environmental distributions of products of this mitigation process (including soluble organic compounds) and pathways of transport of oil and combustion product chemicals into the water column and eventual deposition into sediments, even in deeper waters.
- Products of in situ burning need to be further assessed for both atmospheric emissions and chemicals in the water column under slicks subjected to in situ burning. Of particular concern are chlorinated aromatics (e.g., polychlorinated dibenzodioxins) formed in chloride-bearing seawater or in air at the air-sea interface.
- The high-molecular-weight chemicals produced by photo-oxidation and microbial degradation processes (and perhaps subsequent chemical reactions of initial reaction products) need further elucidation as does the role of such materials in sorption-desorption reactions and bioavailability for lower-molecular-weight constituents of partially weathered and partially biodegraded oil.
- Advanced analytical chemistry techniques and advanced genomic/proteomics analyses should be applied to both field samples and samples from laboratory and mesocosm experiments. Scientists reviewing the literature suggest that results would be more useful/meaningful if both techniques were used for analysis of the same samples. This will maximize collection of rich data sets leading to new discoveries in studies of the biogeochemical cycles/fates of spilled oil.
- Improvements are needed in our understanding of SSDI and its effects on physical processes that influence oil and gas droplet sizes and how these droplets interact with subsequent near-field mixing processes. Future studies should focus on the interactions of gas and oil bubbles/droplets; how large oil droplets evolve to small droplets in both horizontal and vertical plumes; interactions among oil, organisms, and exopolymer substances released by microorganisms (MOS and MOSSFA); and determining whether SSDI reduces exposure of response workers to volatiles at the air-sea interface.
- The experimental designs of laboratory and mesocosm experiments need to be optimized to gain new knowledge of fates and effects (including various sublethal effects) of oil and oil dispersant mixtures beyond the standard acute toxicity testing. Also needed are experiments to disentangle the effects of different chemical substances in oil or dispersants.
- A better understanding is needed of how photo-oxidation products may interfere with the efficacy of dispersants in time and space, of the underlying fundamental physical chemistry governing the efficacy of various dispersant formulations, and of how dispersants impact microbial accessibility to oil at the water/oil interface.
- The utility of existing and newly developed dispersant formulations needs to be improved for various crude oil and fuel oil types and for various climatic regimes and ecosystem types.
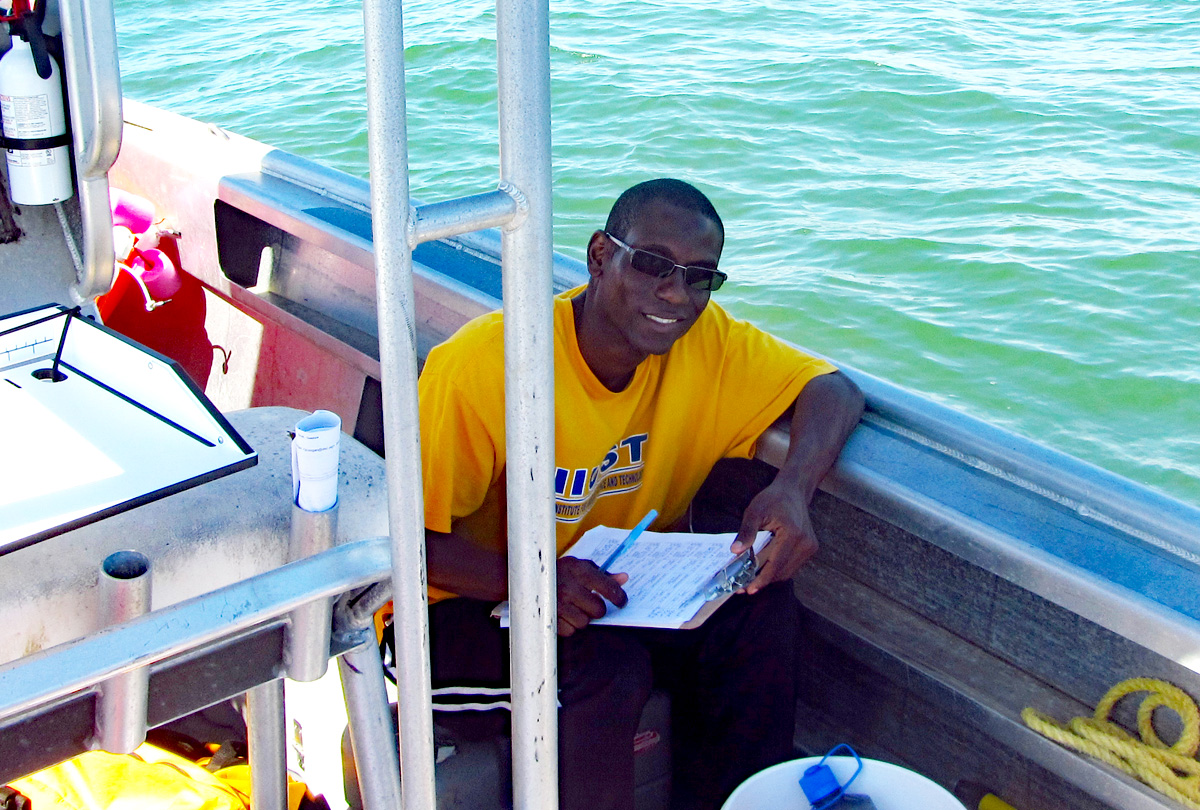
Stephan O’Brien, a PhD student at the University of Southern Mississippi and a GoMRI Scholar, collects water samples at Main Pass, Alabama, for suspended sediment laboratory analyses. Photo credit: Brian Dzwonkowski. > High res figure
|
Biological and Ecological Processes
The DWH oil spill negatively impacted a wide variety of Gulf of Mexico organisms and ecosystems. The primary drivers of those impacts were oil and degraded oil, oil mixed with dispersants, and management responses related to the oil spill. Toxicological impacts upon organisms, generally characterized as lethal and sublethal, were heavily studied over the last 10 years in laboratory and mesocosm settings, as well as in the field, and included field-measured levels of exposure dosages. Impacts identified include immediate and gradual mortality, reproductive failure, and influence on behavioral, olfaction, vision, cardiac function, and gene response. Sublethal effects can be short term, long term, permanent, or even multi-generational (Murawski et al., 2021, and Weiman et al., 2021, both in this issue) and can impact feeding, survival, reproduction, and future generations. Examples of the various documented impacts illustrate common patterns and trends across organisms and/or their environments and show that such impacts might translate to population levels (Murawski et al., 2021, and Halanych et al., 2021, both in this issue).
The development and application of advanced genomic and bioinformatics tools enabled scientists to examine and describe complex microbial communities, identify new species, redefine previously described species and genera, and quantify cellular responses across taxa. These new omics-based technologies and strategies provided an in-depth and comprehensive study of Gulf communities in unprecedented detail and set the stage for integrating genomic data and organismal biology with analytical chemistry and oceanographic modeling. Research communities are now better prepared for new data-driven approaches to monitor and restore ecosystem health.
Advances, Discoveries, and Surprises
Although many instances of organism and ecosystem recovery have been observed since the DWH oil spill (e.g., beaches), in some cases, spill impacts are ongoing and may have caused shifts in the baselines of ecosystem interactions (e.g., deep-sea coral communities). Exposure to polycyclic aromatic hydrocarbons alone may not sufficiently characterize longer-term impacts of oil spills on organisms because environmental impacts were spatially heterogeneous in nature. Rapid recovery tended to occur (to some extent) in high-energy environments, such as marshes and shallow-water regions, whereas low-energy environments, such as the deep-sea benthos, will sustain documented impacts that may last for generations.
Transport of oil spill toxicants throughout the northern Gulf of Mexico was facilitated by organismal activities (e.g., feeding, migration, etc.). Fortunately, microbial communities in the Gulf of Mexico (beaches, marshes, water column, and sediments) were and are uniquely primed for rapid response to oil spills. Some oil-degrading microbes are capable of nitrogen fixation, enabling them to utilize hydrocarbon energy sources without nutrient restriction and providing a strong selective advantage over other species. Moreover, some of these microbial taxa are found to be ubiquitous in oil spills around the globe (e.g., Macondimonas diazotrophica). Bacteria are a primary food source for many organisms and introduce oil-derived carbon into the food web through metabolic pathways. Due to oil droplet sizes that resemble forage bacteria, zooplankton were documented ingesting oil droplets and excreting the oil in their feces, thus depositing oil directly into food webs.
Research has revealed that deeper water communities continue to exhibit impacts. Species abundances in the midwater mesopelagic zone showed major sustained and persistent declines that continued through 2019 (Murawski et al., 2021, in this issue). Reduction in mesopelagic community numbers may have impacted cetacean migration. As described above in the section on Chemical and Biogeochemical Processes, MOSSFA was part of the natural microbial systems’ responses to oil; some deep-sea benthic habitats were smothered by the accumulation of MOSSFA and will take decades to recover. Deep-sea corals showed considerable long-term impacts from spilled oil, but also showed signs of recovery (Halanych et al., 2021, in this issue).
Oiled marshes and associated communities showed unexpected resilience and recovery over time since the spill. However, dolphins in Barataria Bay—where oil entered the marsh—suffered adverse effects, including reduced reproductive success (20% vs. 70%), chronic lung damage, and modified endocrine responses. In addition to the impacts documented for dolphins, novel sublethal impacts of hydrocarbons and dispersants were observed and connected to fish olfaction, metabolic fatigue, immune response, and neural system issues, among others. An associated short-term decrease in abundance of coastal fishes may have accelerated invasion by non-native lionfish.
While many species suffered, several targeted commercial species (including shrimp, crab, and some fish) showed remarkable resilience in the wake of the spill. The closure of commercial fisheries at the time of the spill did not appear to have long-term ecological effects.
Lessons for the Future
Biological systems are highly complex and interconnected. These linkages can aid microbial decomposition of oil or result in damaged ecosystems resetting to a different stable state. In the event of an oil spill, oil-degrading microbes can act as essential and natural first responders, providing critical ecosystem cleanup, transport, and stabilization functions.
Traditional measures of damage to a biological system may not be the best indicators of toxicant impact. Sublethal organismal impacts can turn up in any system or function of an organism’s body. The degree to which toxicological impacts can be placed in meaningful biological or environmental contexts is dependent upon the deployment of analytical chemistry tools.
The DWH spill caused major alterations in microbial community structure and function. Researchers found that novel gene expression, species composition and metabolic capabilities involved in hydrocarbon degradation and nitrogen fixation emerged to dominate ecosystems ranging from the deep sea to salt marshes.
Specific mechanisms behind many organismal and ecological impacts can now be elucidated through the use of genomic tools, which may yield insight into yet unknown long-term implications.
Future Research Needs/Gaps
To complement the many advancements made over the last 10 years, we recommend the following further studies of biological and ecological processes and actions to insure long-term monitoring.
- Conduct long-term monitoring and assessment to provide baseline data for understanding and mitigating the impacts of oil spill toxicants. Biological systems of note are:
- The Barataria Bay, Louisiana, dolphin population, including its reproductive success and endocrine response recovery.
- Mesopelagic communities from plankton to nekton to apex predators, notably the effects of community change and recovery on associated cetaceans and pelagic predators.
- Barataria Bay wetlands, with emphasis on reoiling events.
- Deep-sea benthos and coral habitats that are subject to slow rates of growth and low fecundity.
- Investigate the impact(s) of various components of dispersants on biological systems.
- Conduct longer-term studies on seafood contamination and associated sensory and chemical testing techniques through collaborations between NOAA and the US Food and Drug Administration. Studies should include the investigation of previously unanalyzed oil degradation compounds on marine organisms and associated seafood safety for humans.
- Develop methods to define microbial biomarkers of ecosystem health that will lead to the ability to detect ecosystem disruption, predict risk, guide mitigation strategies, and assess restoration efforts in the face of diverse threats.
- Make progress on translating omics data from observations of novel genes and species into metabolic activity, currently the greatest hurdle to accurately predicting biochemical outcomes.
- Develop more detailed and complete predictive models for biological systems that should integrate biological data and response with chemical, geological, and physical parameters.
- Conduct extensive and regular sampling in diverse ecosystems around the globe to document the normal, or baseline, state of microbial and other communities prior to a crisis in order to plan intelligent mitigation strategies aimed at restoring native communities and their ecosystem functions.
Technological Developments
The severity and unusual circumstances of the DWH incident called for innovative technological developments to mitigate and investigate the fate and impacts of the released material and the physical, chemical, and biological processes involved. The technological developments highlighted in Dannreuther et al. (2021, in this issue) represent only a small sampling of such developments. However, this small representation demonstrates the collaborative and interdisciplinary nature of innovation with application to a wide range of scientific endeavors.
Advances, Discoveries, and Surprises
Technological advancements were applied to obtain measurements of the subsurface dynamics of oil and gas droplets that could then inform estimations of droplet size distribution, a critical component in predicting the transport and fate of a subsurface oil spill. Some measurements were made in laboratory experiments that combined existing imaging techniques with new or enhanced technologies that simulated oil and wave interactions. Other measurements were made in experiments that combined initial blowout conditions with high pressure, mixing forces, and jet stream dynamics. Measurements were also conducted in the field using new deep-sea imaging techniques transported by a remotely operated vehicle to the ocean floor that recorded oil and gas droplets and bubbles rising from natural hydrocarbon seeps. Additionally, the inclusion of oil biodegradation processes in spill simulation scenarios to predict the amount of oil that may reach the ocean surface provided insights that can inform response decisions, especially those related to dispersant use.
Advancements were made in tracking and visualizing the dynamic nature of currents at or near the ocean’s surface, including the potentially different velocities of multiple currents that run just beneath the surface. These discoveries were made possible by new biodegradable GPS-enabled drifters along with drogues, drones and aerostats equipped with cameras, and the novel combination of sensing instruments that mitigated each other’s blind spots. These innovations improved our understanding about the significant transport role that submesoscale processes play and provided data that informs near-real-time transport forecasts during an oil spill.
Several technological modeling developments enabled advancements in quantifying the fate of oil from a marine spill. Such models simulated oil transport via aggregation with floating particulate matter, such as MOS processes, and via oil/particle/mineral interactions that allowed for the inclusion of transport processes not previously accounted for in oil spills. In situ imaging techniques can now distinguish oil and gas droplets in a mixed release that can inform estimations of oil/gas ratios. Additionally, a novel approach that can distinguishing crude oil from non-fossil-fuel carbon sources was applied to estimate the amount of Macondo oil deposited on seafloor sediments.
New techniques were applied to improve the understanding of sublethal impacts from an oil spill on fish. This yielded new insights on the degree to which transcriptomic response to oil is dependent on tissue type. For example, oil-induced impairments to heart cells are linked to a fish’s ability to capture food and escape predation.
Advancements in new dispersant technologies offer the potential for safer and more effective alternatives to existing chemical dispersants used in oil spill response. Scientists revealed that oil/water emulsions created by carbon black particles are more stable and last longer than emulsions created by Corexit. Another discovery showed that clay particles loaded with nontoxic surfactants can be rendered more effective by the use of a stoppering agent that allows for the slow release of the surfactant.
Lessons for the Future
Cross-disciplinary and multi-institutional collaborations can enhance technological developments and increase awareness of, and communications about, the resulting innovations. Such collaborations may include the involvement of spill-response operational scientists directly with academic research projects, which could improve the chance that technological developments and resultant data can immediately inform response decisions during an oil spill. Technological developments designed for use during a marine oil spill can benefit from collaborations with institutes that have facilities for oil-in-water tests, such as the European research organization SINTEF, the SouthWest Research Institute, and the US National Oil Spill Response & Renewable Energy Test Facility Ohmsett. Including academic researchers in spill response preparedness training exercises may improve the academic community’s understanding of the needs and challenges that the response community faces and, thus, can shape academic research and encourage developments to be application focused.
Future Research Needs/Gaps
Innovative technological developments will continue to be driven largely by need and circumstance. Specific suggestions for future development include:
- Continue to advance the processing efficiency of large data sets as the technologies used to acquire those data become increasingly efficient and affordable.
- Continue collaborations with legal authorities, response agencies, and industries that have testing and production-scale capabilities to enable the use of alternative dispersant technologies in large-scale oil spill response.
- Incorporate the latest technological/chemical measurement techniques into safety devices worn by response workers to assess levels of exposure to chemicals of human health concern.
- Increase the use of remotely operated technologies to improve the safety and cost efficiency of conducting in situ biological experiments in the environment, such as using drones to gain access to, and sample, contaminated areas.
- Focus on development of nanoscale assessment technologies for genomics.
- Improve visualization techniques for multiple types of data to help understand and convey the complexity and scale of disasters like DWH.
Socioeconomic and Public and Human Health Issues
The DWH oil spill, according to the National Oil and Hazardous Substances Pollution Contingency Plan, is the only declared spill of national significance in US history. DWH caused significant and lasting adverse impacts on the health and well-being of people and their communities in the Gulf of Mexico region. These impacts compounded and exacerbated the negative effects of Hurricane Katrina and previous disasters in the Gulf, and, in turn, may add to effects of subsequent disasters as well as ongoing threats from chemical pollution, oil seeps, and harmful algal blooms. Studies to date, and those still underway, have shown various adverse mental, physical, and community health effects in some individuals who responded to the spill and/or participated in clean-up efforts, as well as some coastal residents in all five Gulf states (Sandifer et al., 2021, in this issue).
Additionally, the spill had socioeconomic impacts, especially to the tourism and recreational sectors, and those dependent on living resources, such as fishing. These effects also harmed the health and well-being of individuals and communities that were primarily dependent upon natural resources for employment and as a way of life. Residents who were already disadvantaged, low-income, and of minority ethnicity or otherwise vulnerable were most affected. Negative perceptions about the safety of Gulf seafood and its marketability persisted long after data demonstrated its safety according to regulatory protocols in place at that time. These perceptions caused consumers to question the potential for exposure to toxic oil-related chemicals during the spill and clean-up, as well as from seafood consumption. Parents were concerned about children as they played on beaches that may have been contaminated with oil. Some residents reported more stress from navigating the legal and claims processes following the spill than from the spill itself. The breadth and severity of mental, physical, and community health effects, perceived effects, and anxiety about potential effects were significant and, in some cases, were long lasting.
Advances, Discoveries, and Surprises
During initial post-DWH public health data collection, except for information available about US Coast Guard personnel (Rusiecki et al., 2018), researchers were faced with the reality that baseline health and socioeconomic data against which to compare spill effects were woefully inadequate. As a result, this scarcity of systematically collected and curated health and socioeconomic data made it difficult to characterize individual, community, and societal impacts and to connect outcomes with their causes. The need for ongoing data collection of these sorts was further emphasized when studies revealed that secondary events occur in the periods between major disasters.
This information made it evident that health services are especially important for community members who are not directly involved in spill operations but are vulnerable to indirect spill effects such as social and economic impacts. Unfortunately, well-structured and funded human health services and data collection associated with large oil spills were lacking compared to those for environmental effects. US oil spill response protocols focus on mitigating the environmental pollutant but generally lack formal mechanisms, developed with public input and support, for assessment of impacts on humans and communities and recovery of damages. Furthermore, insufficient involvement of the public in communicating health and safety information resulted in increased anxiety and distrust of authorities.
Lessons Learned
In the event of another disaster, the lessons learned from the DWH oil spill need to be applied to future human health assessment and recovery. Researchers should be aware that large oil spills are associated with a broad range of mental and physical health impacts and may amplify the adverse effects of both prior and future traumas. Such impacts are most pronounced among vulnerable people and those individuals and communities dependent upon natural resource-related industries.
These health impacts occur through both direct contact with spilled oil and indirect exposures to it, such as a spill’s social and economic effects. Also, health studies on oil spill response workers tend to be slow to start and inadequate in duration and depth; health studies for those not involved in spill response are even less adequate. While there is an increasing awareness of the need to deal with indirect health impacts associated with oil spills, structured programs and secure funding to do so are lacking.
Future Research Needs/Gaps
Recommendations for future research and study regarding socioeconomic and public and human health issues are outlined in Sandifer et al. (2021, in this issue). Some of the most critical actions recommended are:
- Implement the health observing system framework (Sandifer et al., 2021, in this issue). This will require participation of a diverse group of health professionals and government, institutional, and private sector entities.
- Collect and analyze health, environmental, and socioeconomic data to identify cause-and-effect links between spills and health outcomes to improve preparation, response, recovery, and damage assessment.
- Develop robust mechanisms for dialogue among oil spill and other disaster preparedness authorities and researchers prior to disaster events.
- Improve toxicological profiles for oil spill chemicals, including dispersants, in humans, prioritizing vulnerable human populations.
- Improve human exposure and effects models and human health risk assessments.
- Substantially increase research on models of human health and socioeconomic effects and integrate them with oceanographic and ecosystem models.
- Increase transdisciplinary research on the human dimensions of oils spills and other disasters.
- Improve personal protective equipment supply and its utilization for responders.
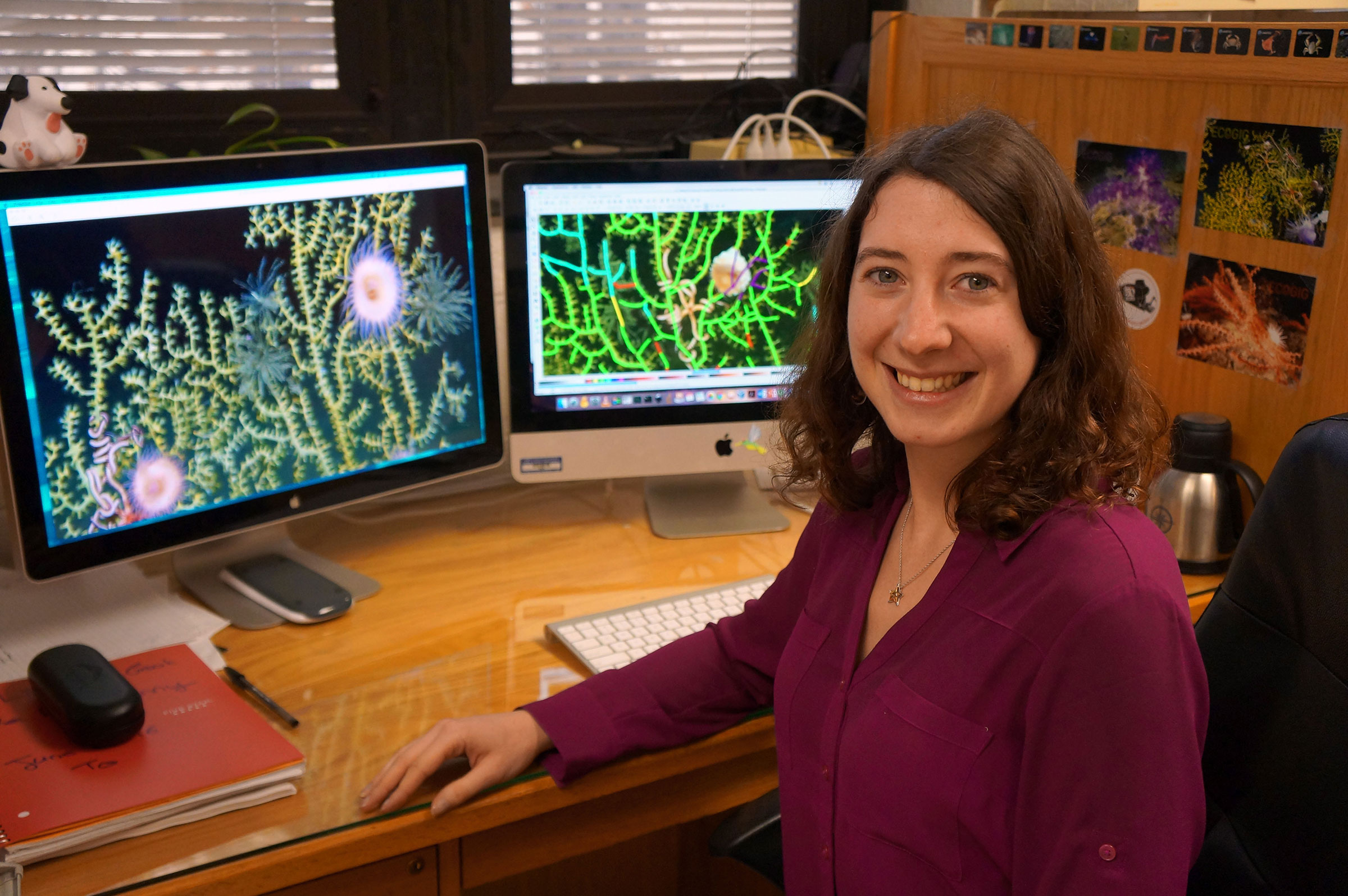
Graduate student Fanny Girard of Penn State, a GoMRI Scholar, digitizes a high-definition image of a coral colony to quantify impacts, growth, and recovery. Photo credit Cherisse DuPreez. > High res figure
|
Preparedness, Planning, and Advances in Operational Response Issues
Planning and preparing for oil spills are key to expediting clean-up and minimizing environmental harm during an actual event, but response leaders are often faced with time-critical and complex options that inevitably create trade-offs (Westerholm et al., 2021, in this issue). Determination of oil fate and transport and associated environmental impacts accurately and promptly is imperative. Over the last 10 years, research helped propel advances in spill response, damage assessment, and quantifying resources at risk, but research gaps remain. The nexus between operational response and research involves many entities, including state and federal agencies, industry, academia, and nongovernmental organizations (Halanych and Westerholm, 2021, in this issue). In future efforts, industry and government should continue to collaborate with academia to improve operational response.
State-of-the-art fate and transport models were developed and utilized during the DWH event to predict landfall and assist strategic deployment of response resources, but these models had limitations. Because the DWH incident was an undersea blowout, the few available three-dimensional operational models were inadequate for capturing droplet formation and circulation at depth, which limited the federal government’s deep-sea blowout oil spill modeling capability. Since the DWH incident, improvements in spill modeling have included the addition of weathering parameters, enhanced three-dimensional capability, faster processing, new algorithms, and more user-friendly displays on a variety of platforms (Westerholm et al., 2021, in this issue). Other modeling needs remain, as there are no fully quantitative models that can simulate oil spill impacts across all the physical, chemical, biological, socioeconomic, and human health knowledge domains.
As a result of the DWH Natural Resource Damage Assessment and GoMRI research, improvements in sample collection, use of genomics, and extensive toxicity testing have all led to a better understanding of the Gulf of Mexico ecosystem (Murawski et al., 2021, and Halanych et al., 2021, both in this issue). Multidisciplinary researchers continue to discuss the nexus between laboratory research and mesocosm studies on toxicity, dispersion, microbial degradation, and other spill impacts and how these should be incorporated into the planning process and environmental trade-off discussions (Halanych and Westerholm, 2021, in this issue). Response decision-makers are typically faced with new and alternative technologies, in addition to dispersants, that require quick evaluation. While there is a process for evaluation, deploying new methods or untested equipment during an emergency can have adverse impacts and increase liability. During the DWH spill response, numerous alternatives were proposed and some, such as freshwater diversion, were utilized. The diversion, which was designed to minimize damage to coastal wetlands by keeping oil offshore, instead demonstrated a trade-off decision that had unintended and severe ecological consequences.
Lessons for the Future
The operational response command structure must continually strive to develop and evaluate new scenarios that can be incorporated into spill planning and exercises. Often, plans and models are based on past assumptions of expected spill impacts (e.g., specific amount and type of oil, specific location, what additional injury might result from response actions). However, more work needs to be done on the few models that currently allow comparative predictive modeling of alternative mitigation scenarios and the expected impacts of using, not using, or combining specific technologies. For example, “what if dispersants or in situ burns are not utilized?” In those cases, the plans and models need to evaluate the expected impacts and recovery times for the oil (and its products) that might now reach nearshore and coastal environments. By updating plans and models, future response leaders can better predict real-time impacts that optimize the deployment of response resources and that allow them to make difficult trade-off decisions on these alternatives.
As research brings new capabilities to bear, the command structure will also need to evaluate these alternatives and incorporate them into their existing spill response plans. This might include introducing microbes to enhance biodegradation (natural and engineered), new dispersant or chemical herding formulations, more efficient in situ burning, uncrewed equipment for sample collection and dispersant applications, and detection and imaging technologies that could safely increase operational windows to a continuous 24/7 response. Communicating these options and engaging the public as early as possible will promote understanding and may have profound benefits for addressing societal concerns and mental health issues.
Prior to a spill, federal agencies must work with stakeholder groups and seek expert opinions that allow for a clear value system relative to oil impacts. These agencies and the command structure during the spill will need to communicate this process more effectively to explain how and why certain decisions were made.
Future Research Needs/Gaps
The ultimate goal of GoMRI was to improve society’s ability to understand, respond to, and mitigate the impacts of petroleum pollution and related stressors of the marine and coastal ecosystems, with an emphasis on conditions found in the Gulf of Mexico. As highlighted in this issue, GoMRI has significantly increased the ability for the response community to react, respond, and mitigate future spills; however, there are several areas that require additional research, focus, and funding:
- High-resolution oceanographic models do not yet exist for all areas where spills might occur. A system is needed that can quickly employ a high-resolution operational regional model, nested within the existing larger-scale operational models. Future models must also capture spatially variable and non-isotropic diffusion as well as vertical transport associated with MOSSFA. While simplified MOSSFA models can currently be incorporated into operational models, improved parameterizations of processes such as subgrid-scale diffusion are still lacking.
- Fate models can be improved by incorporating biodegradation and photo-oxidation and their effects on emulsions, oil solubility, and toxicity, including sublethal impacts. This also includes modeling the expected interaction of response alternatives, such as dispersants, for these processes and any changes that would occur over time in microbial degradation or photo-oxidation.
- Quantifying and reducing the uncertainly of forecasts, as well as including other domains of knowledge such as biological ecosystems, socioeconomics, and human health, are critical for development of accurate models. GoMRI has explored a framework for assessing a quantitative model that simulates oil spill impacts across these domains, but the model itself does not yet exist.
- Future models will need to be scalable and incorporate combinations of all response options over time, evaluating and comparing the expected benefits and impacts while accounting for the predicted and often changing environmental factors associated with specific spill locations.
- As seen in DWH, when restoration alternatives are implemented, long-term impacts on marine and estuarine habitats must be considered and monitored.
- Modeling restoration alternatives should include even more complex processes associated with nutrient overloads and land use management, climate change impacts, natural erosion, and future energy development. Based on the DWH incident, it is imperative to develop new classes of predictive numerical models to explore the effects of planned large-scale projects, such as freshwater diversions. Such models would integrate estuarine, coastal, and shelf hydrodynamics, such as riverine forcing, and incorporate ecological processes and outcomes, thus allowing for better predictions of both the short-term effectiveness as a spill countermeasure and the expected outcome of long-term habitat restoration projects.
Conclusions
The human tragedy and ecological impacts of DWH will persist for years/decades to come and will never be forgotten. Yet, this tragedy has taught those involved to be safer, smarter, and better prepared for managing offshore resources. The subsequent funding by BP, which led to the establishment of GoMRI, demonstrated that an independent, science-based research program can successfully bring diverse groups of scientists together to achieve common goals. This cross-pollination of different disciplines yielded advances and real-world impacts that benefit understanding of the Gulf of Mexico as well as other locations where marine oil exploration and extraction occurs. The past 10 years of effort and attention have reinforced how important and understudied coasts and the open ocean remain. It was imperative to use the best science available to fully understand environmental impacts and determine the appropriate means to ameliorate those impacts through restoration. Filling critical data gaps, some of which are highlighted above, will be necessary to support better future restoration and response decisions while also establishing new baselines that require long-term studies (Wiesenburg et al., 2021, in this issue).
GoMRI research has built a foundation of knowledge and tools that will enable scientists to play key roles in guiding future oil spill response and Gulf of Mexico science with data-driven strategies. The creation and preservation of a permanent data archival system (Zimmermann et al., 2021, in this issue) will prove invaluable in the future and support the new mindset of open-source data in science. Government and private supporters of science should be encouraged to require that future research grants, awards, and contracts submit collected data to open-source data portals.
GoMRI presented a rare opportunity to successfully assess and follow spill impacts and synthesize findings across academia, industry, and government. The post-DWH cooperation and information sharing between empiricists, modelers, and decision-makers should be a guiding example for future large-scale events. The relationship between research funding and academia’s connection to operational decision-makers should continue beyond GoMRI in order to close gaps, evaluate new opportunities, and bring the best available science to spill response plans and operations.
After 10 years and a $500 million investment, GoMRI scientific research yielded findings that will benefit the Gulf of Mexico ecosystem for decades. New species were discovered and understanding of ocean currents and microbial degradation of oil was significantly advanced. This new knowledge helps us better understand the environmental impacts of released oil in the environment and how spilled oil moves through the Gulf of Mexico, with many complex effects on both the environment and citizens of the region.
As a closing statement, the authors wish to express their gratitude to all established and early career researchers who undertook such excellent work. The support and partnerships within industry, academia, the Gulf of Mexico state governments, and federal agencies greatly aided the research. Lastly, we acknowledge the sacrifices, as well as the contributions, of those who lived through the DWH spill, as well as those we have lost.
Acknowledgments
The authors would like to thank the cast of thousands who participated in GoMRI and who made this groundbreaking research possible. Credit is due to the vision of the principal investigators and consortia directors, the meticulous attention to detail of the project managers, the creativity of the outreach coordinators, the technical flexibility of the data managers, and all others who contributed to this 10-year initiative. Special thanks go to the lead authors of the other 14 articles that comprise this special issue, who took on this important task with grace and enthusiasm. Lastly, we would like to thank the entire GoMRI Research Board for their superb leadership, foresight, guidance, and friendship over the last 10 years.