Introduction: State of Play in the United States
In the United States, offshore wind is an important component of the renewable energy goals being set by coastal states. At the end of 2019, offshore wind capacity commitments summed across states was 19,968 MW by 2035. In some states without offshore wind-specific targets, such as California and Hawai‘i, 100% renewables portfolio standards and carbon reduction policies are driving the creation of new offshore wind lease areas (DOE, 2019). This has led to an increase in proposed projects (i.e., wind farms) in recent years, particularly in the northeastern United States, with seven plans currently under review and an additional eight plans expected by the summer of 2021. All of these projects are proposed to be built within the 16 areas of ocean bottom currently leased to developers. In total, these areas cover 6,880 km2 located off of the US Atlantic (Figure 1) and Pacific coasts.
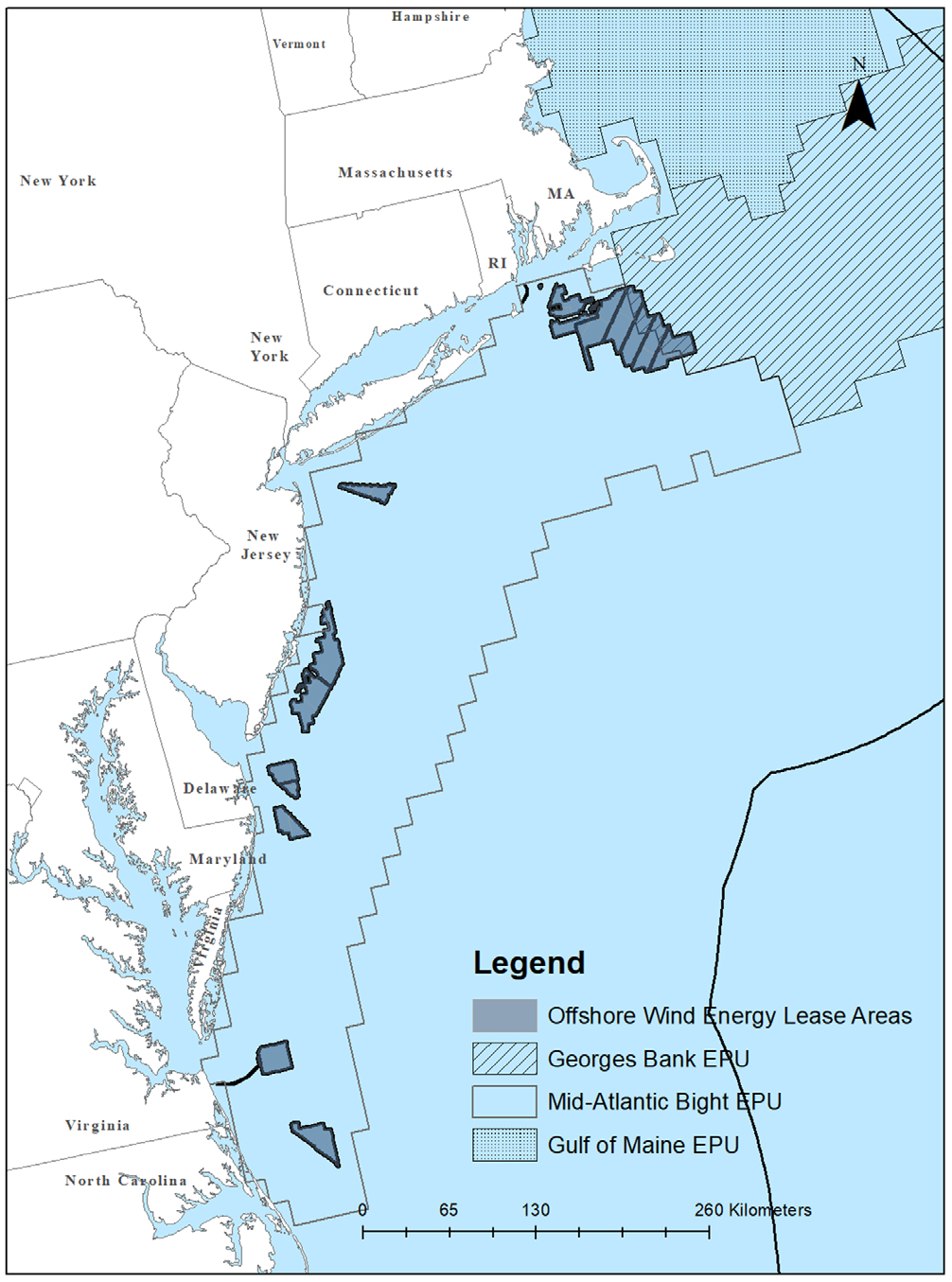
Figure 1. Map of the 16 currently existing wind energy lease areas in the Northeast US Shelf Large Marine Ecosystem. Fishing activity overlaps with the entirety of the Ecological Production Units (EPUs) presented on the map. Total area of leases = 6,880 km2. > High res figure
|
Wind development in the offshore zone will overlap with fisheries that contribute important economic, recreational, and cultural resources to American society. The initial focus of US wind energy development is in the northeastern region of the country where commercial and recreational fishing were underway long before the nation formed. The historical and cultural importance of commercial fisheries in the region is reinforced by its economic importance, with $3.7 billion in value added to the economy in 2016, supporting over 260,000 jobs (NMFS, 2018). Recreational fishing, which was initially a form of subsistence, has grown in economic importance in the region over the past 50 years, with over $5.3 billion added to the economy in 2016, supporting more than 69,000 jobs (NMFS, 2020). Marine fisheries are managed both by the states (Atlantic States Marine Fisheries Commission) and the federal government (NOAA Fisheries and Regional Fishery Management Councils). Both state and federal management are legally structured to have stakeholders among the decision-makers, with management decisions implemented by state and federal governments.
Wind energy development is a new ocean use in the United States that competes with longstanding activities. It will have significant impacts on fishery activity, fishery resources, and fishery science and management (BOEM, 2020), and trade-offs among these sectors can be explored in an integrated ecosystem assessment framework (Levin and Lubchenco, 2008). For offshore wind in the United States, the Bureau of Ocean Energy Management (BOEM) is the lead agency for the approval of projects in federal waters. However, multiple state and federal agencies have authorities for different aspects of permitting and responsibilities for reviewing and assessing the potential impacts of projects. Sites to be developed have been chosen based on multiple factors to reduce competition, including mitigation of viewshed impacts, avoidance of areas used for military operations and sensitive radar systems, and availability of wind resources. The planning process does not automatically exclude competing use areas like fishing grounds; rather, it mandates that decisions include “consideration” of fishing activities (Energy Policy Act of 2005 Section (p)(4)(J)(ii)). How fisheries are considered under this requirement is largely left to the discretion of the Department of the Interior. This has led to a great deal of confusion and concern among fishery participants because there is no threshold of impacts to commercial fishing activities that would affect offshore wind energy siting and development decisions. Lack of fishery stakeholder participation in the wind development decision-making process contrasts with the participatory governance structure of fisheries management (Figure 2), which has been in place for decades and clearly defines the involvement of stakeholders. Fishery stakeholders have been included to some degree on a state-by-state basis; for example, a state purchasing energy from a development may include fishers in their state in the wind development process. However, wind energy development sites are in federal waters and may impact fishers and fishing communities from multiple states. This disconnect further amplifies the differences between the governance processes of fisheries and wind energy development.
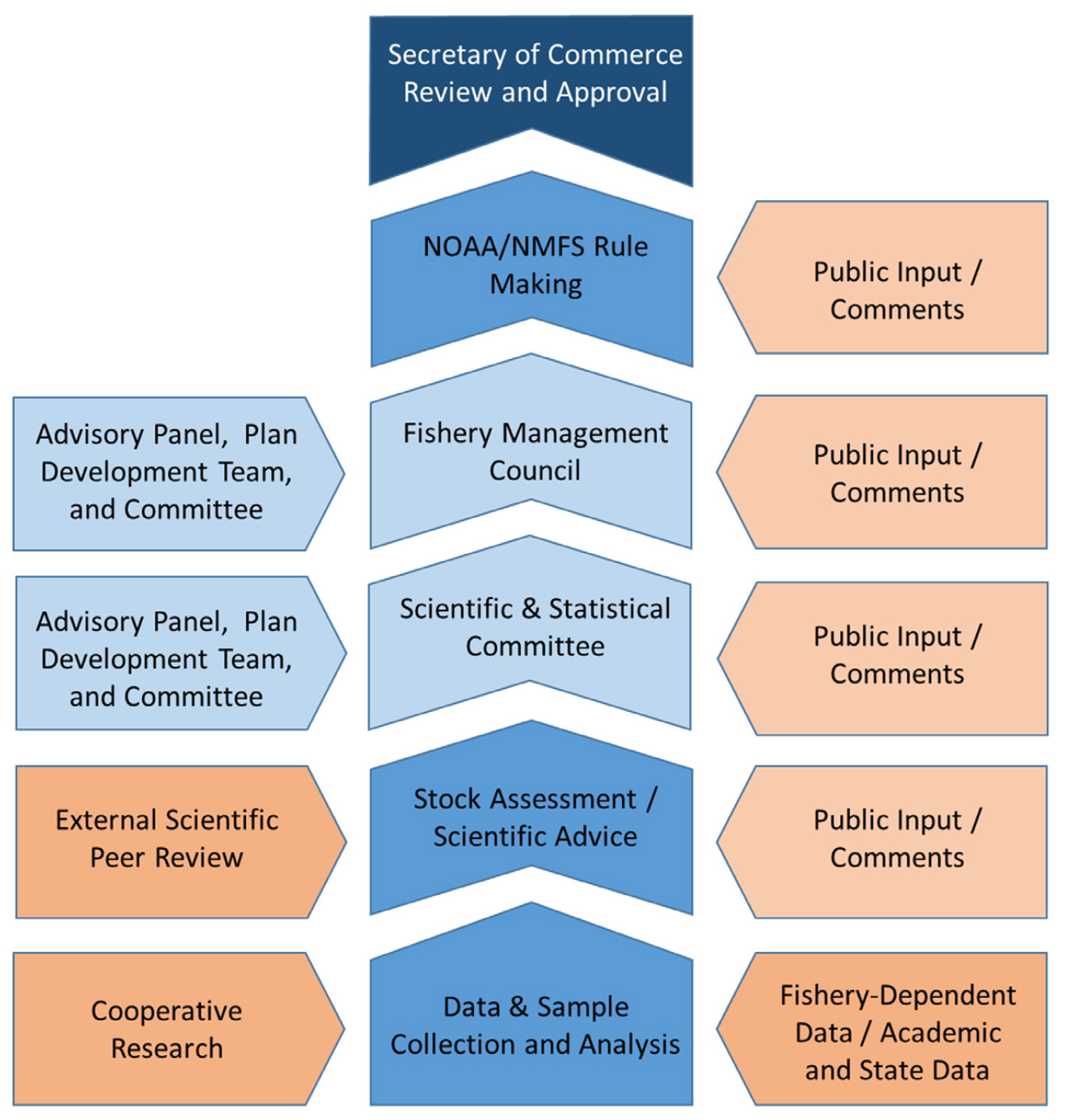
Figure 2. Overview of the participatory governance structure of fisheries management in the United States. Dark blue: National Marine Fisheries Service (NOAA/NMFS) and Department of Commerce-led activities. Light blue: Regional Fishery Management Council-led activities. Orange: Opportunities for engagement in the science and management process. > High res figure
|
Our objective in this paper is to broadly define the potential interactions between offshore wind energy development and fishing, with a focus on the Northeast US Shelf Large Marine Ecosystem (NEUS-LME). We take a regional perspective, recognizing the regional scale of wind energy development, fishing activities, and fisheries management. From this summary of interactions, we identify challenges to and opportunities for the goal of coexistence of offshore wind energy development and fishing activities. In this regard, the goal is renewable energy and sustainable seafood—our future depends on both.
Effects of Offshore Wind Development on Marine Ecosystems in the United States
Interactions with Ecological Communities
Ecological interactions can occur during each phase of offshore wind development, from pre-construction site assessments to decommissioning (Birchenough and Degraer, 2020). Multiple stressors are associated with offshore wind development, and a comprehensive review of each of these stressors is provided by separate papers in this special issue. Table 1 describes the potential effects for eight species from the NEUS-LME that are emblematic of the challenges faced in this system. These eight species were chosen to be highlighted because of their importance to key ecosystem functions, their significance to the commercial and recreational fishing communities, and/or because of their status as endangered or critically endangered species. While these species may be unique to the NEUS-LME, they have analogs in ecosystems around the world. Adverse effects are expected for some species, others are expected to benefit, and the outcome for others remains uncertain due to lack of information. Currently, few stressors are understood with a high degree of certainty either because so few studies have been conducted or because those that have been conducted have shown conflicting results. This underscores the urgent need for further research to understand each of these stressors and their integrative effects in the marine environment.
Table 1. Potential interactions of offshore wind development with species representing major groups from the Northeast US Shelf Large Marine Ecosystem (NEUS-LME). > High res table
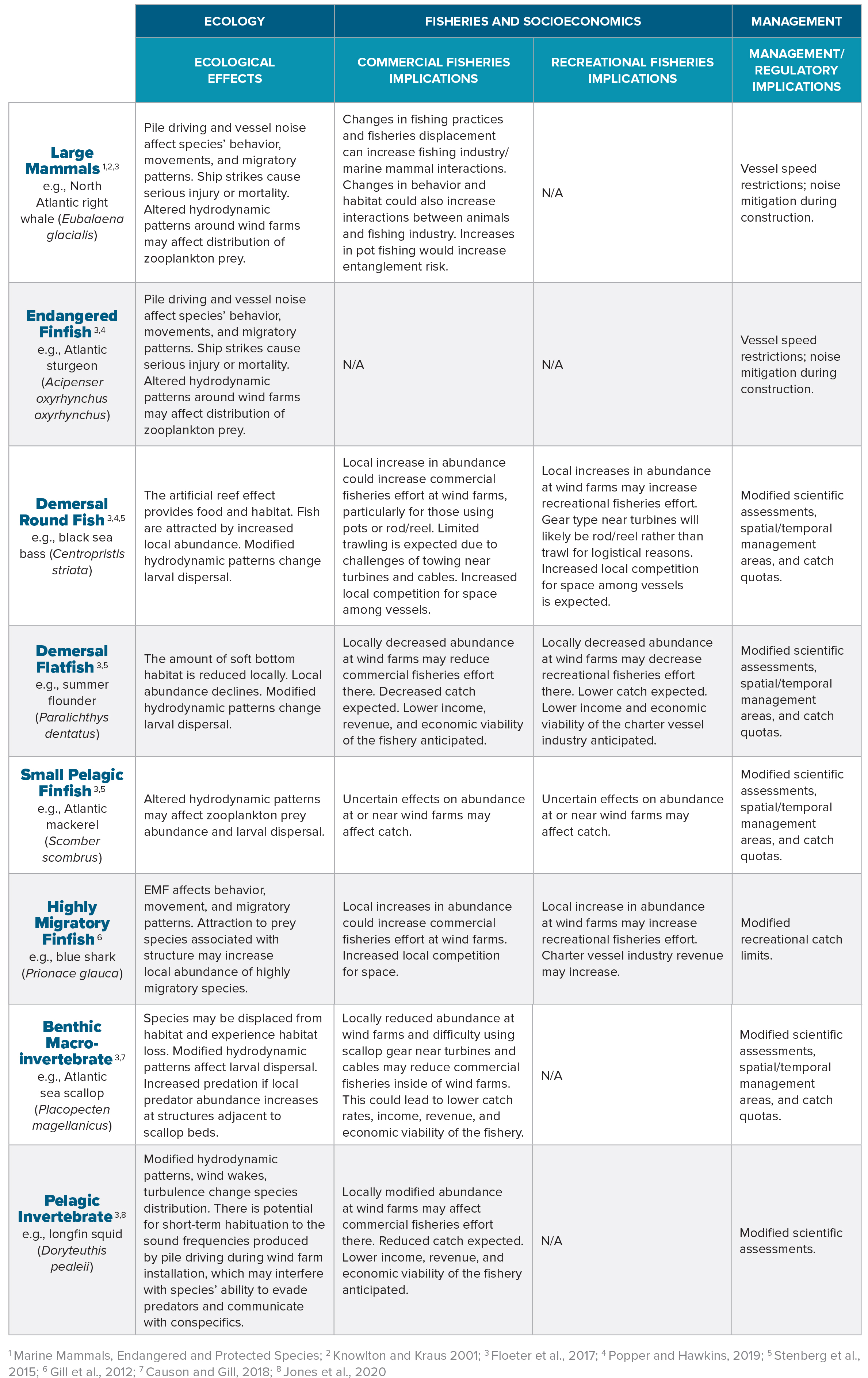
|
Interactions with Human Communities: Fisheries and Socioeconomics
Fishing communities have numerous concerns associated with offshore wind, including increased risk of collision with fixed structures (turbines and cables) and non-fixed structures (other vessels and gear) due to changes in routing patterns and increased vessel traffic, as well as potential for interruption of fishing by wind development activities, regulatory uncertainty (new fishing restrictions), and diminished economic opportunity resulting from competition between commercial and recreational fisheries (Gray et al., 2016). Fishers have also expressed concern about impacts to traditional fishing practices due to predicted shifts in resource distribution that may require significant changes to current fishing methods and locations.
Although wind projects in the United States are planned to be open to commercial fishing, there are many logistical challenges associated with operating vessels in and around fixed and non-fixed wind energy structures, particularly vessels using mobile fishing gear. The challenges include difficulties with navigation, physical obstruction, traffic, safety, gear loss, and possible insurance changes. Together with shifts in target stock distribution, these potential obstacles may make wind facilities de facto fishery exclusion areas, potentially leading to redistribution of fishing effort and stock-wide changes in quotas or catch limits. Such exclusions will not only have direct effects on excluded vessels but will also have indirect effects on vessels as well as ecosystems elsewhere as displaced effort will increase competition in remaining fishable locations. Fishing cessation and its effect on the benthic community could also have significant feedback effects on population indices (Roach et al., 2018) and “multiplier effects” that ripple through coastal businesses, communities, and the downstream seafood trade. Understanding these issues requires gathering finer-scale fisheries data (i.e., landings per tow), which are often proprietary, as well as gaining a better understanding of the economic value of seafood once it enters the supply chain. Indeed, offshore wind developers and management authorities have attempted to quantify direct effects on commercial fishing as part of compensatory mitigation programs and/or environmental effects analyses (e.g., Livermore, 2017; Fugate, 2019). However, these assessments are controversial and do not include the full suite of fishing interests impacted by development. Within some sectors of the recreational fishing community, there is a perception that offshore wind facilities will enhance fishing (Hooper et al., 2017). While it is well documented that offshore structures are utilized by the recreational fishing community (Smythe et al., 2018), few publications show that these enhancements are an actual benefit to fish at the population level.
Fisheries impacts in the United States are expected to differ from those observed in Europe because of disparities in fisheries infrastructures, markets, and ecosystem conditions. For example, the fleets most affected in the UK consist of smaller day-boat vessels, and there is more uniformity among target fisheries and locations among vessels from a given port. In contrast, the US fleet is highly mobile and more diverse with regard to vessel size and locations fished.
Interactions with Fisheries Management
Under current US law, regional fishery management councils will continue management of fisheries in wind energy areas, which are sited in federal waters. Changes in fishing locations, effort, and gear types may require additional management actions by councils. In addition, competition between commercial and recreational fishing may have implications for fisheries management that need to be addressed by the councils. The Mid-Atlantic and New England Fishery Management Councils, which manage fisheries in the Northeast, have developed management measures for application to specific areas (e.g., Habitat Closed Areas, Gear Restricted Areas, Special Management Areas), and changes to the management of these areas or the implementation of new site-specific regulations could be called for in and around wind energy development areas.
Much of fisheries management involves establishing catch levels that are based on stock assessments. A number of data sources are used in stock assessments, including scientific fishery resource surveys, and these surveys will be impacted by wind energy developments. In the Northeast United States, a number of scientific surveys overlap with wind development areas and represent more than 315 years of cumulative survey effort which are supported by dedicated NOAA ship and aircraft resources (Table 2). Information gathered from these surveys represents some of the world’s most comprehensive data on marine ecosystems (e.g., Despres-Patanjo et al., 1988; McClatchie et al., 2015). The surveys support fisheries and protected species assessments and management actions, ecosystem-based fisheries management, and regional and national climate assessments, as well as a number of regional, national, and international science activities. Offshore wind development will impact these scientific survey operations and consequently the scientific and management products produced for a wide variety of users. Within offshore wind areas, survey operations will be curtailed or eliminated under current vessel and aircraft capacity limits, safety requirements, and assessment protocols. Without robust investment in a plan to adapt these data collection and analysis programs to offshore wind development, the programs will suffer from survey bias, a reduction in information, increased uncertainty in stock assessments, and resulting poorly informed management decisions. When uncertainty is introduced into stock assessments, management decisions are less well informed, and the likelihood of inappropriate management actions increases. Poorly informed management actions could lead on the one hand to overfishing of stocks and on the other hand to underfishing of stocks. Both have significant economic impacts on commercial and recreational fishing industries.
Table 2. Characteristics of core NMFS scientific surveys in the Northwest Atlantic and their overlap with offshore wind development. Overlaps are the percentage of survey strata that overlap with wind energy leases and wind planning areas as calculated in a spatial analysis. SNE = Southern New England. MAB = Mid-Atlantic Bight. > High res table
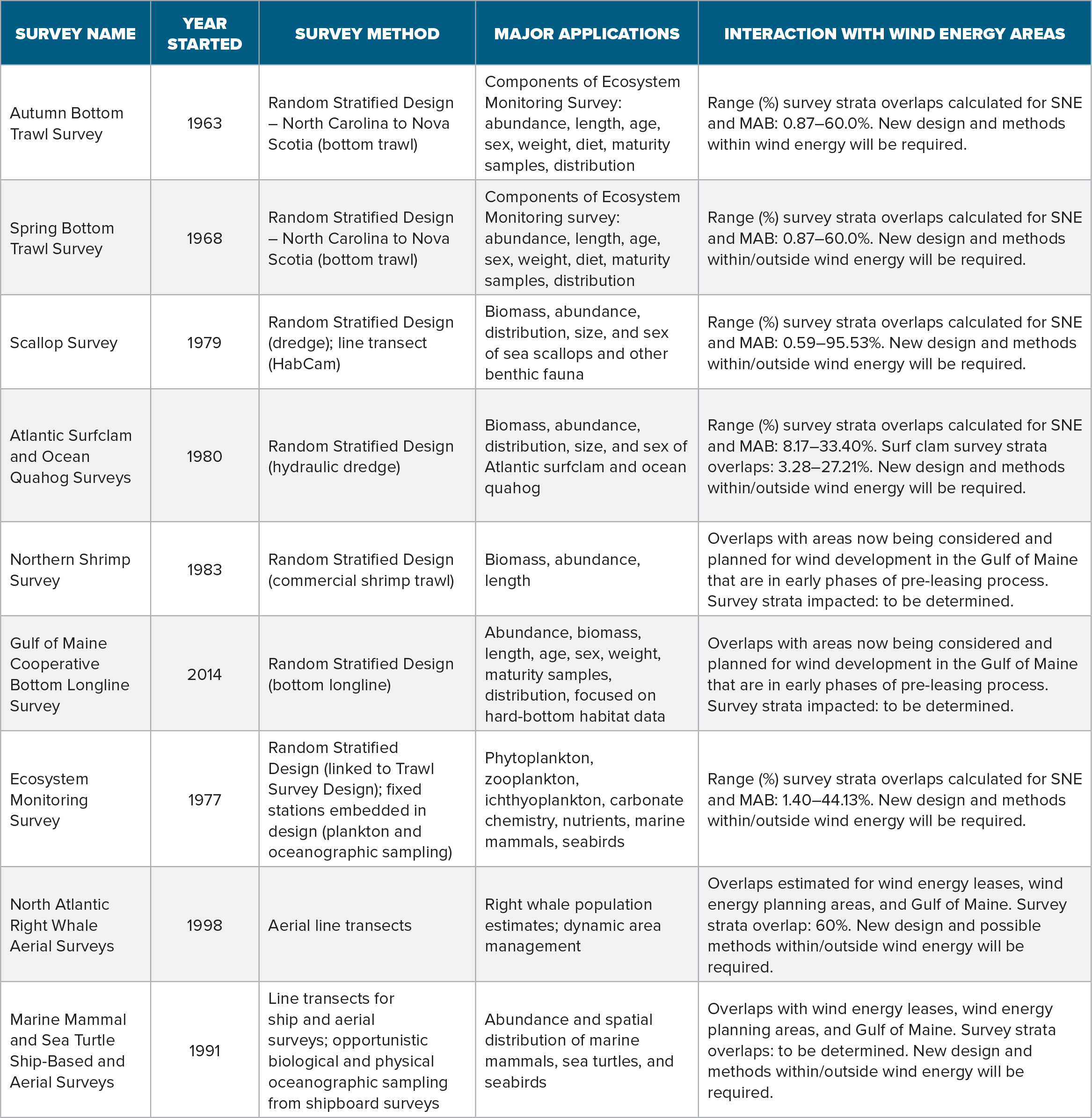
|
The Challenges
Keep Learning on Pace with Development
The rate of offshore wind construction and technological advancement is extremely fast-paced. At the same time, numerous analyses are needed in order to understand biological and human interactions with offshore wind. One of the greatest challenges is to keep these analyses on pace with the offshore wind development because they provide vital information for permitting and management decisions. From a regulatory perspective, challenges include a high number of project plans, multiple intersecting state and federal mandates, contrasting missions of cooperating federal agencies, and budget limitations imposed on cooperating agencies to address these challenges. From a science perspective, reconciling the time and resources needed to identify and conduct scientific investigations at ecosystem and development scales with the timetables of wind projects is a huge challenge. Keeping science on pace with development will require an adaptive approach in which learning occurs simultaneously with development and our tools for monitoring and mitigating effects are adapted as our knowledge base grows and evolves.
Assessing Cumulative Impacts
Grappling with the effects of a single wind project is challenging. Understanding the cumulative impacts of multiple wind projects at full build-out in combination with other past, present, and reasonably foreseeable stressors operating over several decades is much more difficult. Cumulative impact analysis (CIA) for offshore renewable energy development (Willsteed et al., 2018) is particularly arduous because of the high levels of analytical complexity and uncertainty associated with offshore wind interactions with marine ecosystems. In the United States, multiple offshore wind projects are slated for construction over the next decade, and operations will continue for an additional 25+ years per project (BOEM, 2019). Climate-driven changes in the distributions and abundances of marine species, variability in the energy and seafood markets, and evolving wind and fishing technologies (Nye et al., 2009; BOEM, 2019) lead to highly uncertain outcomes in cumulative impacts analyses; the focus tends to be on a maximum impact scenario. Evaluating existing CIAs to identify strengths and weaknesses of current practices (Willsteed et al., 2018) and the development of new methodologies such as those implementing risk-based approaches (Stelzenmueller et al., 2018) are moving CIAs toward practicable applications.
Potential Effects of Evolving Turbine Technologies
Fixed foundation technologies are currently most feasible in waters ≤60 m deep, yet more than 58% of the offshore wind energy in the United States occurs beyond this depth (Beiter and Musial, 2016). The most promising technology for deepwater offshore wind is floating turbine systems that are anchored to the seabed using mooring lines and anchors. Floating turbines are connected to each other by an intra-array network of subsea electrical cables and to land by sub-bottom export cables (Statoil, 2015). In the United States, a demonstration-scale floating wind turbine was deployed in the Gulf of Maine between 2013 and 2014, and deployment of a full-scale turbine off the coast of Monhegan Island, Maine, is planned. In addition to the Gulf of Maine, floating technology is also currently being explored for use off the US West Coast and Hawai‘i.
Compared to fixed foundation technology, floating wind facilities will encounter a different set of marine species and fisheries in deeper waters and will create a different set of stressors. For instance, as with fixed foundation facilities, floating facilities are still likely to limit or exclude fishing vessels with towed gear, stir up sediment into the water column during burial of export cables, and support epifaunal growth on associated structures. Floating offshore wind may also present new effects related to the mooring or cabling systems that are not yet fully understood (Statoil, 2015). However, the noise associated with pile driving would be eliminated, and floating wind systems could also allow for greater flexibility in siting because of their broader depth allowances.
The Opportunities
Cross-Sectoral Collaboration
The development of offshore wind in the NEUS-LME brings enormous challenges, but it also creates equally immense opportunities for collaboration and innovation. Collaborative efforts among federal agencies, the commercial and recreational fishing communities, and the wind industry are already underway. In March 2019, NOAA, BOEM, and the Responsible Offshore Development Alliance (RODA) entered into a 10-year memorandum of understanding (MOU), setting the stage for collaborative work between regional commercial fishing communities and federal regulators on areas of mutual interest. RODA, a commercial fishing industry coalition with members from North Carolina to Maine as well as from the west coast of the United States, formed in response to the considerable challenges fishers have faced in their efforts to be effectively involved in offshore wind energy planning. The organization is working with NOAA Fisheries and BOEM to enhance engagement with the commercial fishing industry in the offshore wind development process, identify optimal approaches for incorporating fishing expertise into the planning and development process, and support regional research and monitoring efforts that include fishers’ ecosystem knowledge. As one early effort under the MOU, the three entities are collaborating to develop a synthesis-of-the-science report and workshop to compile and integrate existing scientific knowledge about offshore wind and fisheries interactions. In addition, RODA is also formally engaged directly with a group of eight wind developers to create a standing Joint Industry Task Force in order to improve communication between the two industries and bring commercial fishing industry expertise to bear on major issues of concern such as wind project siting and layout design. Together, these collaborations create opportunities for applying improved marine planning techniques so that wind projects better match expectations of US fishing communities. They also aspire to create new opportunities for research and collaboration, to spur innovation, and to develop new mitigation strategies.
Regional Coordination of Scientific Study
There is a critical spatial mismatch between wind project-level data (hundreds of square kilometers) collected by developers and the potential uses of these data, uses that include studies of impacts on (1) ecological communities (10 m to thousands of meters), (2) regional fisheries resources, (3) fishing communities and seafood industries (local, state, and regional), and (4) the scientific enterprise that supports fisheries management (Table 3). These spatial mismatches are further complicated by the wide geographic ranges of fisheries resources (up to thousands of kilometers) and the regional nature of fisheries management structures. Thus, a regionally coordinated framework is greatly needed for collection, analysis, and sharing of information from offshore wind facilities. Such a framework is essential for addressing conflict between fishing communities and developers. A regional approach to science would standardize monitoring methods (sampling gear, experimental design, spatial and temporal scales that more closely match the resource, and reporting standards) within a region to make data comparable within and among projects. Individual regions could incorporate regionally specific studies into the larger research framework, and the research questions and their answers would complement one other in much the same way that puzzle pieces fit together to give a full picture.
Table 3. Examples of pathways through which offshore wind development could modify ecosystems and the expected scales of direct effects on fisheries resources, fisheries/socioeconomics, and fisheries management. Spatial scales are categorized as local (within the footprint of the wind farm or adjacent neighborhood), regional (at the scale of managed populations/stocks, which may be thousands to tens of thousands of meters from wind farms), or ecosystem (more than 100,000 meters from a wind farm). > High res table
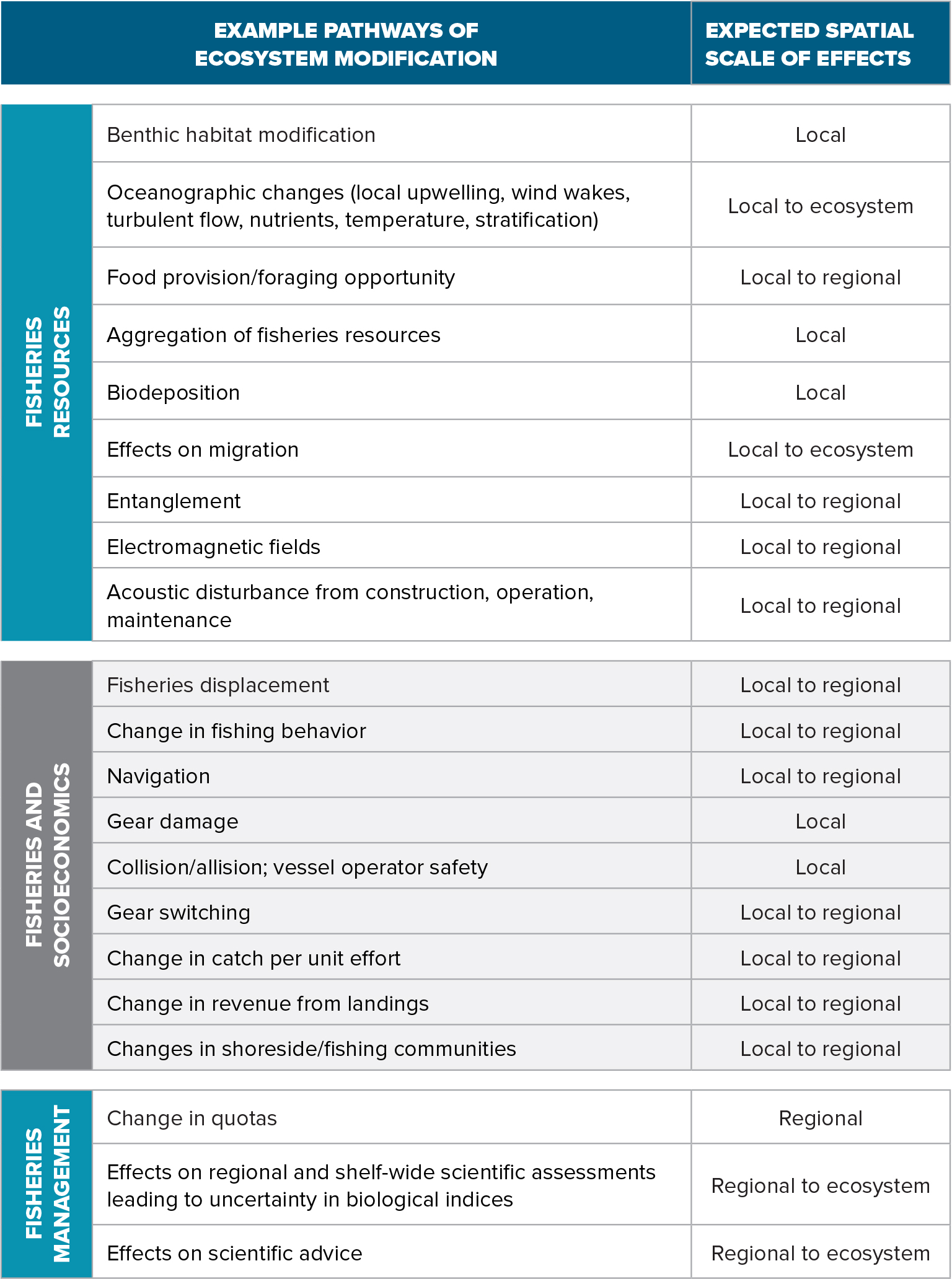
|
Collaboration will be the cornerstone of regional science efforts. Through RODA, commercial fishing communities have opened a dialog with wind developers to address conflicts between the two industries and suggest opportunities for cooperative research. The Responsible Offshore Science Alliance (ROSA), a newly established regional science structure in the United States for which RODA was a founding member, will take a lead role in coordinating the development of a regional science framework (https://www.rosascience.org/). ROSA’s overarching mission is to coordinate and deliver the best available scientific products and information necessary to address offshore development, fisheries management, and ecosystem health. By facilitating regional coordination, ROSA will foster collaboration across disciplines, work toward standardizing methodologies, and establish best practices for scientific study both within and among regions. Existing regional fisheries monitoring collaborations such as the Northeast Area Monitoring and Assessment Program (NEAMAP, 2003), a cooperative state and federal program that facilitates data collection and sharing, are excellent examples of integrating fishing industry platforms and fishers’ expertise and can provide models to advance offshore wind and fisheries science.
Learning from and Building Collaborative Bridges with European Partners
Offshore wind has been generating energy for European nations for more than 20 years (Olsen and Dyer, 1993), and those nations continue to face many of the same challenges related to science-based monitoring, commercial fisheries interactions, and fisheries management that the United States is now confronting. Major research and monitoring programs have been underway at individual European wind facilities for several years (e.g., Degraer et al., 2019), and this work is increasingly making its way into the peer-reviewed literature in the forms of reports on individual research studies, research syntheses, and policy papers (e.g., Gray et al., 2016; Causon and Gill, 2018; Roach et al., 2018, Methratta and Dardick, 2019). To foster collaborations between US and European colleagues going forward, the International Council for the Exploration of the Sea (ICES) has recently convened the Working Group for Offshore Wind Development and Fisheries (WGOWDF). The central purpose of the WGOWDF is to bring the multidisciplinary expertise of its membership to bear on these issues (Box 1) in synergy with other existing ICES working groups, including one focused on Marine Benthal and Renewable Energy Developments.
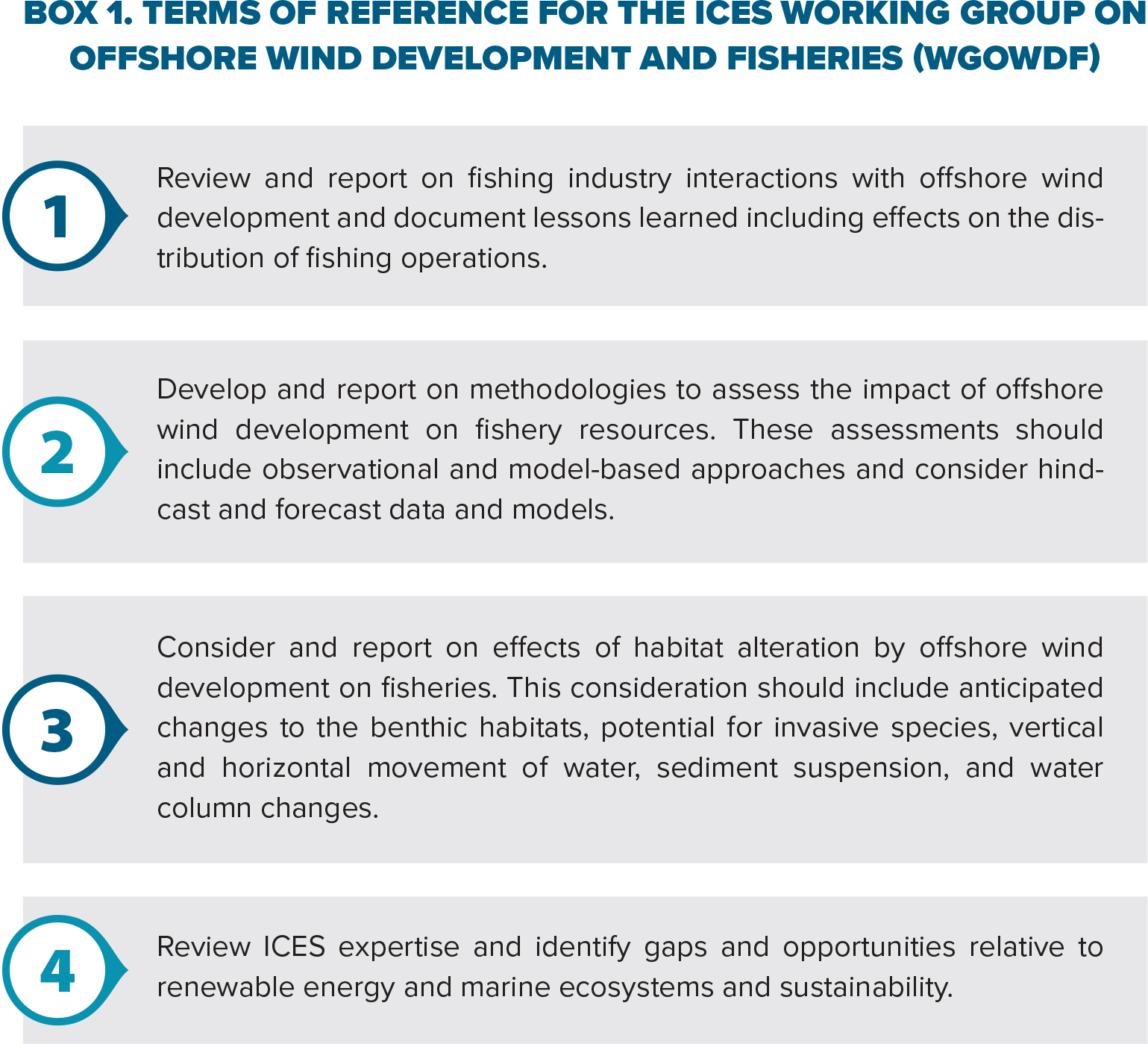
|
Learning from Previous Experience
The Block Island Wind Farm (BIWF) is the first operational offshore wind facility in US marine waters. Commissioned in late 2016, BIWF is a 30 MW demonstration-scale project consisting of five 6 MW turbines located in state waters 6 km off the coast of Block Island, Rhode Island. The BIWF experience provided an opportunity to learn about how offshore wind interacts with both ecological and human communities. First, it illustrated the importance of early and effective industry-to-industry dialogue in the context of a comprehensive and inclusive marine spatial planning process for clarifying respective concerns and for identifying ways to minimize conflict, mitigate impacts, and generate options for mutually beneficial cooperation (McCann et al., 2013). Next, the BIWF experience demonstrated how the commercial fishing and offshore wind industries could work collaboratively to design ecological impact studies, including both a cooperative groundfish trawl survey and a ventless trap survey for American lobster (Homarus americanus) (Lipsky et al., 2016). In addition, the BIWF experience provided an opportunity for the first studies of offshore wind effects in a US ecosystem, including studies of the acoustic environment during pile driving (Amaral et al., 2019), sediment suspension and deposition during cable laying (Elliott et al., 2017), and establishment of the post-construction benthic community (LaFrance Bartley et al. 2019), as well as effects on the flatfish community during construction (Wilber et al. 2018). Lastly and perhaps most importantly, with few unambiguous findings from these studies, the BIWF experience demonstrates the ongoing challenges in attaining a clear understanding of ecological effects. This is due in part to low statistical power and high uncertainty in individual studies. But the larger issue illuminated by these research efforts is the high degree of complexity in the interactions between wind development and environment and just how critical rigorous study designs are for understanding them.
The United States also has a long history with other offshore industries, such as the oil and gas industry, which can be further explored. For example, the fishery liaison/fishery representative framework that was developed in the UK (FLOWW, 2014) and adopted in the US offshore wind energy sector had some of its earliest roots in the Joint Oil and Fisheries Liaison Office (JOFLO) established for the oil and gas industry offshore southern California. With regard to environmental effects, analogs concerning the artificial reef effect post-construction and the effects of decommissioning structures can be brought to bear on understanding potential life-cycle effects from offshore wind (Birchenough and Degraer, 2020).
Need for Innovation
Successfully surmounting the challenges brought by offshore wind development will require innovation across several arenas, including monitoring technology, experimental design, and cooperative research. The difficulty of sampling with traditional trawl or dredging gear is driving innovation in new monitoring methods that include using environmental DNA (eDNA), both aerial and underwater autonomous vehicles, remotely operated vehicles (ROVs), digital aerial photography, automated sensors, stereo camera technology, artificial intelligence, and machine learning (Thomsen et al., 2012; Kresimir et al., 2016). Innovative experimental designs such as the before-after-gradient (BAG) method are being explored. BAG can offer many advantages over the traditional before-after-control-impact (BACI) design by explicitly incorporating spatial heterogeneity and improving the ability of a study to detect changes from baseline (Methratta, 2020). Innovation in cooperative research is spurring discussions on the use of appropriate gear and on how, when, and where to sample. It is also catalyzing collaboration among vessel operators to improve real-time data collection and to create new uses of owner-collected data. If approached deliberatively, future opportunities may exist for gear modification, vessel or marine radar improvements, or improvement of port infrastructures.
Integrated Ecosystem Assessment as an Organizing Approach
Ecosystem-based management provides a framework for balancing the ecological and human dimensions of offshore wind through consideration of how ecosystem components, including humans, interact with management sectors across the entire ecosystem (Levin and Lubchenco, 2008). As an operational approach to ecosystem-based management, Integrated Ecosystem Assessment is a multi-step process that permits the evaluation of cross-sector trade-offs (Samhouri, et al., 2014; Figure 3). Approaches to including wind facility development into an Integrated Ecosystem Assessment framework in the United States would benefit from examining previous efforts at wind facilities elsewhere to link functional diversity with ecosystem services (Causon and Gill, 2018), to identify ecosystem indicators (Raoux et al., 2019), and to develop ecosystem simulation models (e.g., Pezy et al., 2018).
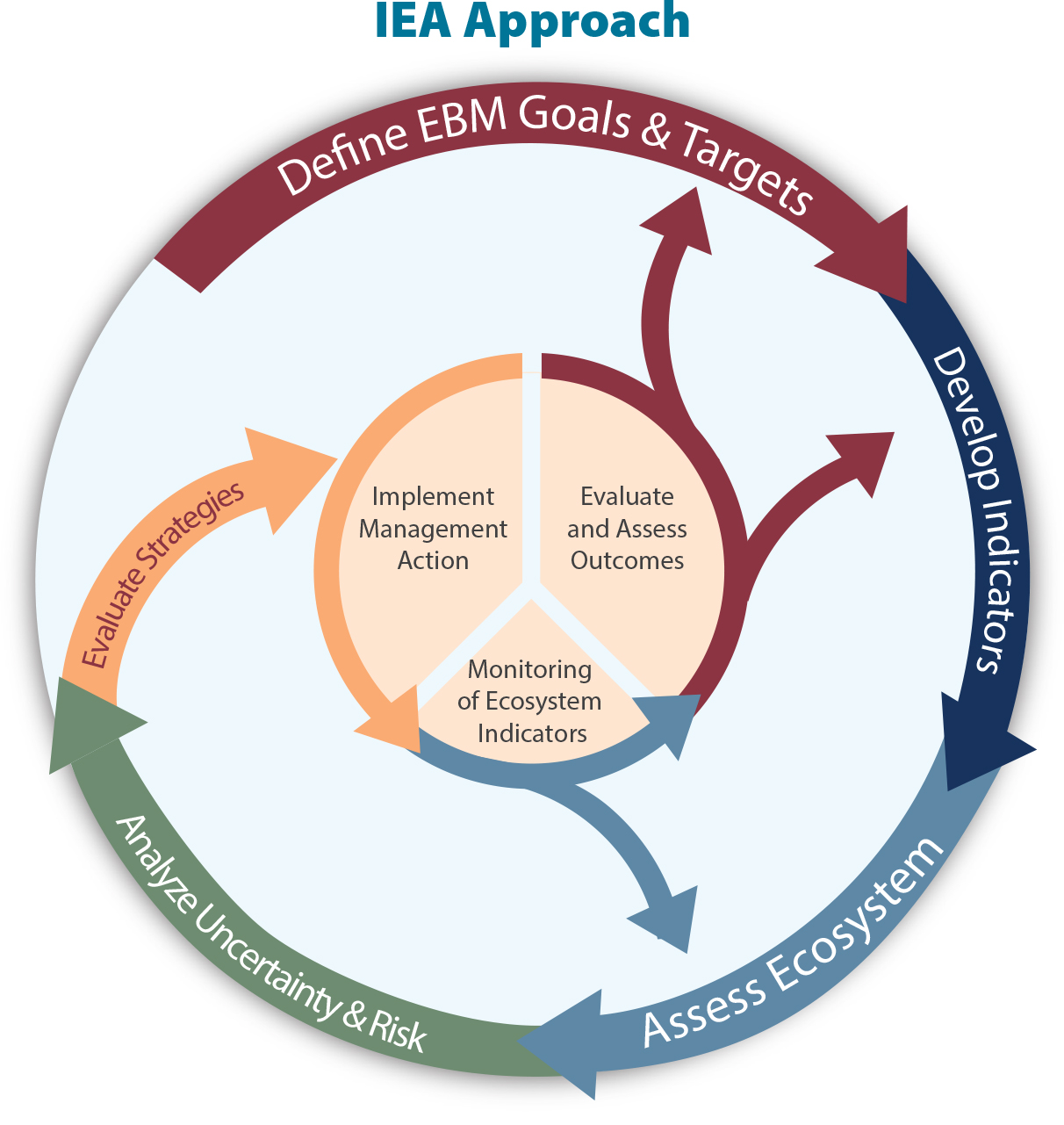
Figure 3. The NOAA Integrated Ecosystem Assessment (IEA) approach, a multi-step process that permits evaluation of cross-sector trade-offs. EBM = Ecosystem Based Management. From NOAA IEA Program, https://www.integratedecosystemassessment.noaa.gov/national/IEA-approach, Samhouri et al., (2014). > High res figure
|
Conclusions and Recommendations
Renewable energy and sustainable seafood are both integral elements of a sustainable ocean economy and will certainly both be needed to support the future of society. The spatial overlap between these two industries in the offshore zone creates not only many challenges but also many opportunities. Achieving coexistence will involve an all-of-the-above approach that incorporates a combination of collaboration, regional approaches, and innovation. To that end, we make the following recommendations:
- Continue to advance cross-sectoral collaborations.
- Co-design methods and approaches with the fishing community in coordination with wind energy developers to address specific areas of conflict, collect enhanced spatially referenced fisheries data, and create a common framework for mitigating adverse impacts to fishing communities.
- Develop regional approaches to advance the best possible science that utilize standardized methods and an accepted set of best practices for designing impact studies.
- Adapt existing fisheries resource surveys to wind development in order to continue to deliver the highest quality scientific advice.
- Develop means to integrate local-scale monitoring with regional and shelf-wide scientific assessments.
- Continue to innovate in the arenas of cooperative research, monitoring technology, and experimental design.
- Continue building opportunities to learn through domestic and international collaborations that develop operational information products and methods.
- Advance an Integrated Ecosystem Assessment framework that includes offshore wind in the evaluation of trade-offs and cumulative impacts.
Acknowledgments
We thank L. Hice-Dunton, F. Hogan, L. Johnston, and the members of the NOAA Wind Team, including D. Christel, C. Nachman, W. Gabriel, J. Hoey, and A. Silva for their ongoing dedication and the many conversations that continue to move this work forward. We thank D. Christel and two anonymous reviewers for critically reviewing and providing comments on this manuscript. Acknowledgment of the above individuals does not imply their endorsement of this work; the authors have sole responsibility for the content of this contribution. The views expressed herein are those of the authors and do not necessarily reflect the views of the Department of Commerce, the Department of the Interior, or their sub-agencies. All authors reviewed and approved of this manuscript prior to its publication. We thank BOEM and DOE for organizing and funding this special issue of Oceanography and for the opportunity to publish this paper.