Introduction
The complex coastlines and narrow passages of the Indonesian seas provide the only pathways for inter-ocean exchange of Pacific and Indian Ocean tropical waters, known as the Indonesian Throughflow (ITF; Figure 1; Gordon and Fine, 1996, and references therein). The ITF varies at intraseasonal to interannual timescales, driven by regional and remote forcings from the Pacific and Indian Oceans, which set up a pressure gradient and sea level differences between the two (Wyrtki, 1987; Susanto and Song, 2015; Sprintall et al., 2019). Water, heat, and salt fluxes measured within the ITF have been found to be a significant factor in the global ocean thermohaline circulation. Hence, the ITF may impact climate variability of both the Pacific and Indian Oceans and beyond. Temperature and salinity changes in the Pacific and Indian Oceans may influence dynamics of the Asia-Australia monsoon, the El Niño Southern Oscillation (ENSO), and the Indian Ocean Dipole (IOD) (Bryden and Imawaki 2001; Lee et al., 2002; Sprintall et al., 2019).
“The results of our research in the Alas Strait serve as an example of how collecting and analyzing oceanographic data are essential to the management of environmental issues facing mining companies located near coasts.”
|
Although the ITF has been measured over the last three decades, these data have only been collected in the passages of major inflow, the Makassar Strait and the Lifamatola passage, and outflow, the Lombok, Ombai, and Savu Straits and the Timor passage (Arief and Murray, 1996; Murray and Arief, 1988; Murray et al., 1990; Gordon et al., 1999, 2010, 2019; Molcard et al., 2001; Susanto and Gordon, 2005; Sprintall et al., 2009, 2019; van Aken et al., 2009; Susanto et al., 2012; Wang et al., 2020). Only recently have minor inflow (Karimata Strait) and outflow (Sunda Strait) passage measurements been collected (Fang et al., 2010; Susanto et al., 2010, 2013, 2016; Wang et al., 2019; Wei et al., 2016; 2019; Li et al., 2018). However, none of these measurements include the ITF in the straits along the Nusa Tenggara (Lesser Sunda) island chain from Bali to Timor (i.e., Bali, Alas, and Sape Straits). Hence, their contributions to inter-ocean exchange between the Pacific and the Indian Oceans have not been recorded.
This paper presents newly released current velocity and temperature measurements collected in the Alas Strait. These measurements were made from November 2005 to February 2007 using bottom-mounted acoustic Doppler current profiler (ADCP) and thermistor arrays. The Alas Strait, located between the islands of Lombok and Sumbawa, is one of a series of straits within the Nusa Tenggara island chain that connects the internal Indonesian seas (the Banda and Flores) to the Indian Ocean. The water depth at the northern entrance of the Alas Strait is approximately 400 m, and there are some small islands on either side off of Lombok and Sumbawa. Along the north-south strait, the water depth decreases slightly from 180 m to 125 m, with a sill at 95 m depth, where the mooring is located. The strait’s average width is ~15 km. The mooring location (width 16.8 km) is slightly north of the narrow constriction, placed to avoid a ferry route that connects Lombok and Sumbawa (Figure 1).
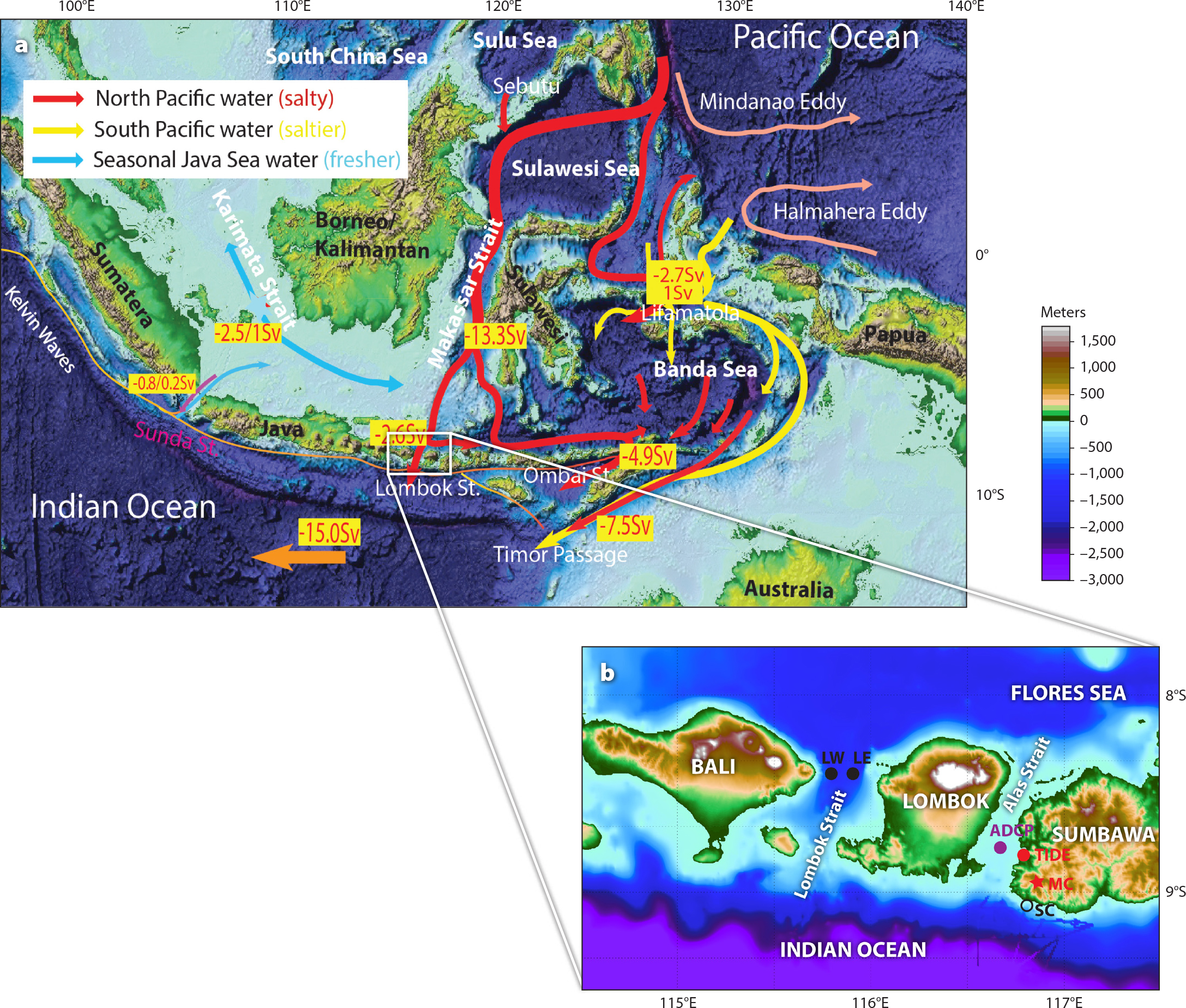
Figure 1. (a) Indonesian Throughflow (ITF) pathways (Susanto et al., 2016). (b) The Alas Strait study area is located between Lombok and Sumbawa islands. The purple circle in the middle of the Alas Strait identifies an acoustic Doppler current profiler (ADCP) mooring. The red circle denotes a tide gauge station. The red star locates the Amman Mineral Nusa Tenggara mining company and meteorological station. The open circle (SC) south of Sumbawa is the location of the Senunu canyon. LW and LE in the Lombok Strait indicate the west and east moorings, respectively, of the International Nusantara Stratification and Transport (INSTANT) program. > High res figure
|
The main objective of the field measurements made by the Environmental Division of the Amman Mineral Nusa Tenggara (PT AMNT; previously PT Newmont Nusa Tenggara) mining company was to understand and assess oceanographic conditions in the Alas Strait in order to ensure the effectiveness of their submarine disposal of mine tailings. As required by the Indonesian government, detailed oceanographic assessments must be made before permission is granted to dispose of mine tailings in Indonesian waters. We use these newly released data to attempt to fill the gap in oceanographic measurements from previous studies of the ITF. We present our findings on the dynamics of current and temperature variability in the Alas Strait, its contribution to ITF transport, and heat-flux variability from the Pacific into the Indian Ocean. We also describe an interesting application of oceanographic measurements.
Field Measurements and Analysis
As part of the PT AMNT mining company’s environmental monitoring program, oceanographic measurements were collected in the Alas Strait using a subsurface mooring, CTDs, and underway ADCPs. Current measurements in and near Senunu Canyon, where the tailings were to be disposed, were collected using a bottom-mounted ADCP mooring, a ship-mounted ADCP, an ADCP/current meter lowered from the ship, and an ocean glider (Waworuntu and Febriana, 2002; Bachtiar et al., 2011). In general, the measurements show that the South Java Current, the South Equatorial Current, the Monsoon Current, and the ITF consistently contribute to the current system southwest of Sumbawa. The ITF plays a crucial role in carrying a fine particulate tailings plume offshore through the Alas Strait, as detailed in a five-year mapping of tailings distribution and footprint conducted by the Oceanographic Division of the Indonesian Institute of Sciences (LIPI).
A subsurface mooring was deployed in the middle of the Alas Strait from November 2005 to February 2007, with recovery and redeployment every three months. The mooring consisted of an RDI workhorse (300 kHz) ADCP, five to nine Star-Oddi Starmon Mini thermistors, and two InterOcean Systems acoustic releases. To adequately resolve velocity to the surface and to reduce mooring drawdown, the mooring was deployed at 95 m water depth, with the ADCP placed 5 m above the bottom where the velocity is close to zero; the uppermost instrument on the mooring was situated below the maximum current. Current velocity and temperature were recorded every 10 minutes. Vertical bins for current profiles were set for every 4 m. Thermistors were placed 10 m apart between 32.5 m and 82.5 m depth.
Other environmental monitoring data collected by the mining company and used for our analysis were drawn from a tide gauge installed at Benete Port (Tide in Figure 1b), where the water level was recorded every 10 minutes, and a weather station located on a peak near the mine pit (MC in Figure 1b), where an automatic wind sensor collected data every 10 minutes. Data from the automatic tide gauge and the weather station were telemetered to the company’s base.
Here, we analyze all of these newly released data sets. Following quality control of the individual ADCP bins, the time series data were filtered and interpolated onto a time base, typically of one hour. The velocity data were then vertically linearly interpolated onto a 4 m-depth grid for each hourly time step, as described in Sprintall et al. (2009) and Susanto et al. (2012). To avoid surface reflection contamination, the first two bins near the surface were removed and replaced with a mean of constant velocity as observed in the third bin from the surface along with continuous shear to the surface. The vertically gridded ADCP velocity time series were then four-day low-pass filtered with a Lanczos filter (Duchon, 1979) to suppress inertial and tidal variability (Sprintall et al., 2009).
Wind, Currents, and Temperature in the Alas Strait
Indonesia is located along the equatorial line that runs between Asia and Australia. Thus, its climate is strongly influenced by the six-month reversal of the Asia-Australia monsoon system (Aldrian and Susanto, 2003). Figure 2a shows the wind speed and direction measured at the mine location (MC in Figure 1b). Figure 2b shows the current velocity at 25 m depth in the Alas Strait. Winds strongly affected the strait’s upper layer flow, especially during the boreal summer (southeast monsoon) from April to October, and even to December, in 2006. Strong, easterly, wind-induced upwelling generated lower sea levels along the southern coasts of the Nusa Tenggara island chain during the southeast monsoon (Wyrtki, 1987; Susanto et al., 2001), creating a stronger north-south pressure gradient and increasing the southward ITF flow (Susanto and Marra, 2005; Iskandar et al., 2009; Ningsih et al., 2013; Siswanto et al., 2020; Wirasatriya et al., 2020). The prolonged anomalous easterly winds extended until December because 2006 was an El Niño year (Niño3.4 positive) and the Indian Ocean Dipole Mode Index was also positive, (Horii et al., 2008; Iskandar et al., 2009), thus inducing more vigorous upwelling along the southern coasts of the island chain and driving a more substantial southward flow (ITF).
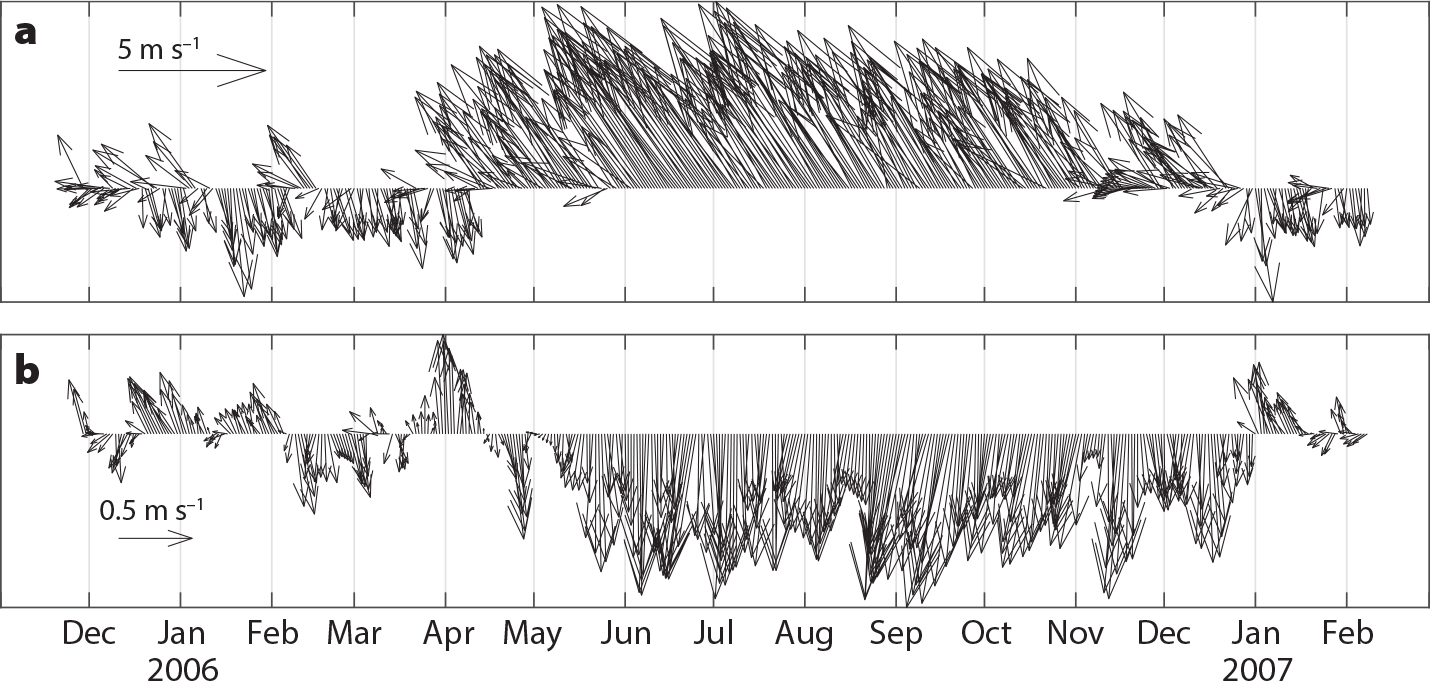
Figure 2. (a) Winds observed southwest of Sumbawa island. (b) Current velocity (m s–1) at 25 m depth in the Alas Strait. A negative sign denotes southward flow toward the Indian Ocean. The upper layer flow in the Alas Strait is strongly affected by wind during the boreal summer (southeast monsoon) from April to October 2006 and even extended until December 2006 due to the El Niño condition (positive NINO3.4 and Indian Ocean Dipole indices). > High res figure
|
Local winds and large-scale remote forcing affect current velocity and temperature in the Alas Strait. To the first order, regional monsoon forcing dominates the velocity profile. Figure 3 shows that north-south velocity measured by ADCP at 0–95 m water depth from November 2005 to February 2007 varies from –1.2 m s–1 (southward) to 0.5 m s–1 (northward). Figure 3a shows the annual and seasonal means in velocity. During the boreal winter (northwest monsoon) of December 2005 to mid-April 2006, northward flow was observed in the upper layer above a southward flow induced by locally wind-driven Ekman currents. This persistent southward flow in the lower layer plays a crucial role in placing the submarine tailing system.
During the boreal summer (southeast monsoon) from mid-April to October 2006, a vigorous southward flow in the upper layer was observed in the Alas Strait. During this monsoon, the combination of lower sea level off the coast of Nusa Tenggara (Wyrtki, 1987, Susanto et al., 2007) and divergence in the Banda Sea (Wyrtki, 1987; Waworuntu et al., 2000; Gordon and Susanto, 2001) drew waters into the Indian Ocean and maximized this upper-layer southward flow. The maximum velocity occurred at 20 m to 30 m depth (Figure 3b). We observe modulation of an interannual signal on top of the intraseasonal and seasonal variability. Starting in the boreal summer of 2006, a positive Dipole Mode Index increased significantly (third largest in the last 30 years after 1997 and 1994), then subsided in January 2007 (Horii et al., 2008). When the easterly wind terminated in early 2007, the regular northwest monsoon reversal flow is observed in the Alas Strait. The presence of the reversal currents is likely due to remote forcing from the equatorial Indian Ocean associated with coastally trapped Kelvin waves as observed in the Sunda Strait (Susanto et al., 2016; Li et al., 2018; Xu et al., 2018), the Lombok and Ombai Straits (Iskandar et al., 2014; Sprintall et al., 2009), and the Sumba Strait (Bayhaqi et al., 2019).
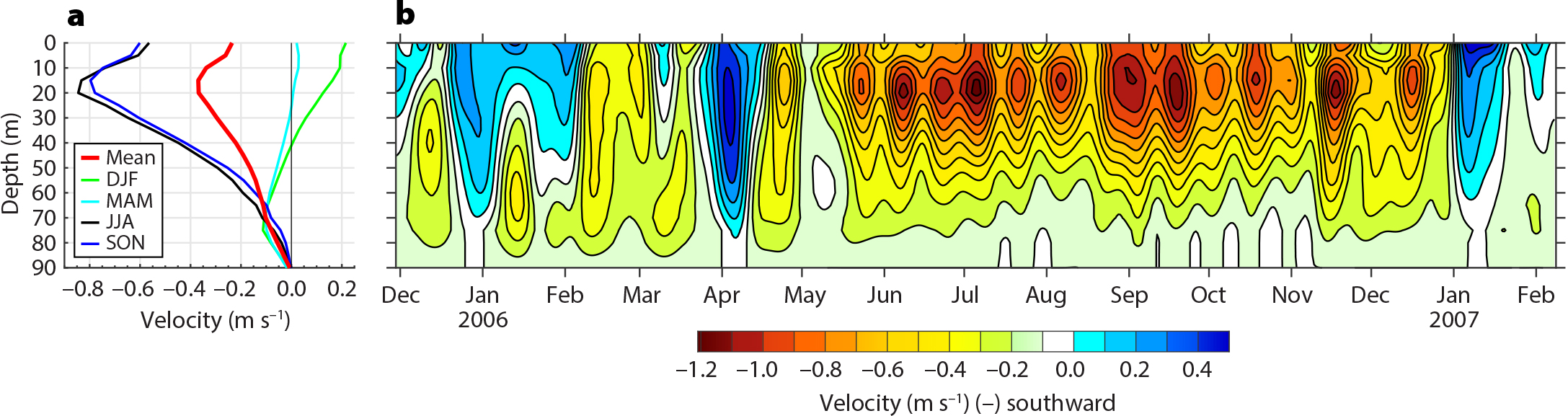
Figure 3. Current profiles observed in the Alas Strait. (a) Seasonal mean and variability of the velocity profile. (b) Velocity profile from November 29, 2005, to February 8, 2007. The negative value denotes southward velocity toward the Indian Ocean. > High res figure
|
Similar to the velocity profiles, temperature variability in the Alas Strait is due to regional monsoon forcing, with interannual modulation associated with ENSO and IOD events. Figure 4 displays temperature measurements acquired with five to nine thermistors attached to the mooring rope between 30 m and 85 m depth. The temperature profile appears to follow the velocity profile. During the 2006 boreal summer and half of the boreal winter (to December 2006), cooler temperatures were associated with the southward flow, while warmer water during the 2005 boreal winter to mid-April 2006 and January–February 2007 was related to northward flow from the Indian Ocean (Figures 3 and 4).
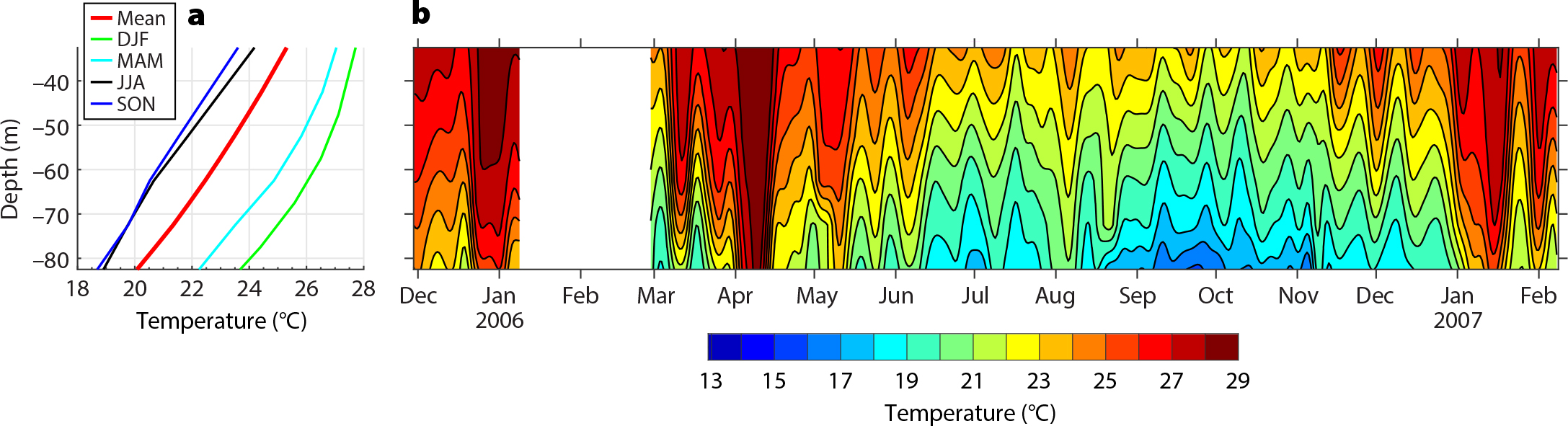
Figure 4. (a) Seasonal mean and variability of temperature profiles observed in the Alas Strait. (b) Temperature profile (°C) from November 29, 2005, to February 8, 2007. > High res figure
|
One of the results of the Alas Strait measurements is observation of the dominant intraseasonal variability on top of the seasonal variability in both ocean currents and temperature. The intraseasonal variability exhibits periods of 13–17 days, 25–30 days, and 70–90 days, and includes reversal flows (northward toward the internal Indonesian seas) during the boreal winter and spring. The 25–30 day and 70–90 day intraseasonal variability are likely associated with coastally trapped Kelvin waves that are generated in the equatorial Indian Ocean and propagate along the southern coasts of Sumatra and the Nusa Tenggara island chain (e.g., Iskandar et al., 2005, 2014). The higher frequency signals (13–17 day period) observed in the Alas Strait during boreal summer were also observed in the Lombok and Ombai Straits (Iskandar et al., 2014). However, there is little energy in the currents at these periods in the equatorial Indian Ocean; thus, these higher-frequency signals, whose maximum velocity occurs at 20–30 m depth (Figure 3b), are likely locally generated and possibly associated with nonlinear interactions between semidiurnal lunar and solar tidal constituents (S2 and M2) and (S2 and N2), which generate fortnightly (period of 14.7 days) and monthly tidal signals (period of 27.5 days; Ray and Susanto, 2016, 2019). Indeed, harmonic analysis of Benete tide gauge data (figure not shown) shows strong lunar and solar semidiurnal components, near fortnightly and fortnightly signals. In situ tidal mixing measurements using a microstructure profiler are needed to validate this finding.
Throughflow in the Alas Strait
The Alas Strait throughflow was calculated with the procedures described in Sprintall et al. (2009) for the Lombok Strait, Gordon et al. (2019) and Susanto et al. (2005, 2012) for the Makassar Strait, and Susanto et al. (2016) for the Sunda Strait. In principle, to estimate the transport within any strait, the mooring point observations need to be extrapolated across the strait with a no-slip condition at the sidewalls. At each bin depth, along-strait velocity is assumed to be uniform across the strait, with zero flow in the last 1 km bin nearest to the sidewalls (i.e., Sprintall et al., 2009; Susanto et al., 2012). The choice of extrapolation schemes (i.e., linear or cubic spline) may generate uncertainty in estimating total integrated transport. Ideally, we should have repeat cross-sectional measurements of ocean currents to reduce uncertainty; however, for various reasons (high fishing activity, ferry traffic, and ship-time constraint), this ideal approach is not always possible.
Given that the Alas Strait is narrow and its along-strait velocity is much larger than its across-strait velocity, we used the only cross-strait velocity data available as a guide for extrapolating data from the mooring site to the sidewalls. Total volume transport is calculated by multiplying the along-strait velocity by the strait’s cross-sectional area at each bin depth and then integrating to the full depth. Figure 5 shows the variability of Alas Strait (red line) and Lombok Strait transport (blue line; the transport is based on the mean of the Lombok west and east moorings from Sprintall et al., 2009) and mean sea level observed at the Benete tide gauge station. The 2006 annual transport was –0.25 ± 0.24 Sv toward the Indian Ocean, varying from 0.4 Sv in early April 2006 to –0.75 Sv in August 2006. Stronger southward throughflow during the boreal summer compared to the boreal winter was evident and was manifested in the mean sea level recorded by the tide gauge. Higher sea level and clearer intraseasonal variability were observed during the northwest monsoon, and low sea level and less apparent intraseasonal variability during the southeast monsoon. Although the annual mean transport through the Alas Strait is low, the seasonal variations are large: ~30% of the Lombok Strait even in reversed direction. This means that the Alas Strait transport serves a double role in the total ITF transport into the Indian Ocean: it enhances the total ITF during the boreal summer and reduces the total ITF during the boreal winter.
Alas and Lombok transport also exhibit intraseasonal variability. At times they are in sync, as they were during the boreal winter from December 2005 to April 2006 (Figure 5). However, during the boreal summer, intraseasonal events evident in the August and October 2006 Lombok transport do not appear in the Alas data. Instead, the Alas transport displayed higher frequency signals (fortnightly and monthly) during that period, which may be associated with nonlinear interactions between lunar and solar semidiurnal signals (Ray and Susanto, 2016). On top of intraseasonal and seasonal variabilities in transport, there is also interannual modulation. The prolonged anomalously early winds in 2006 associated with El Niño and a positive Dipole Mode Index extend the southward flow until December 2006 (Horii et al., 2008). These interannual events may suppress the intraseasonal signals originating from the equatorial Indian Ocean.
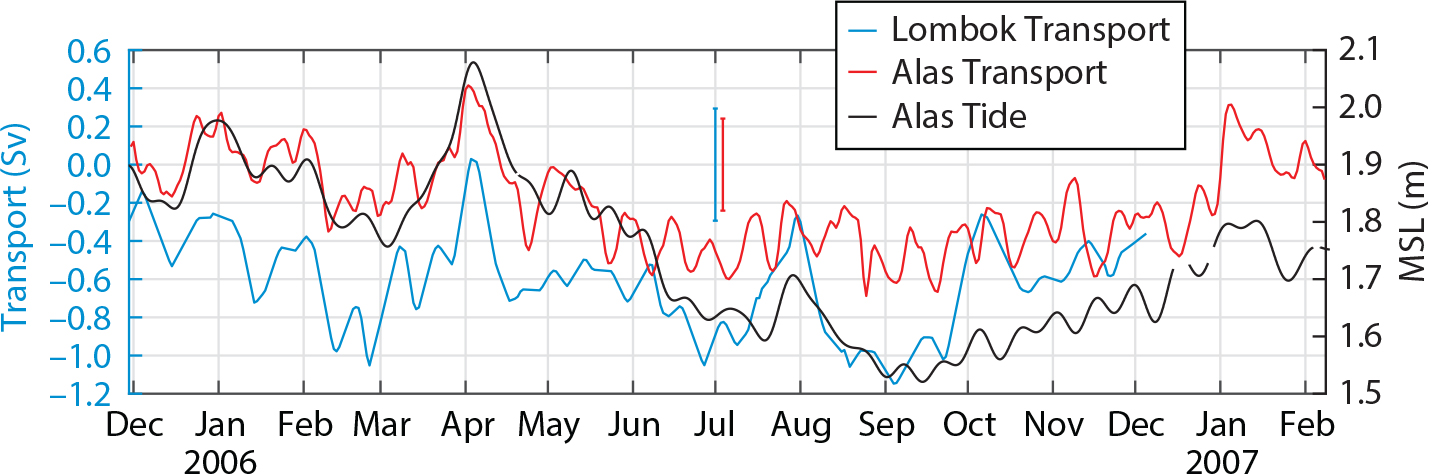
Figure 5. Throughflow transport in the Alas Strait (red line) from November 2005 to February 2007 compared to that of the Lombok Strait (blue line; average transport derived from west and east INSTANT moorings, Sprintall et al., 2009). Vertical lines (blue and red) are the standard deviations of Lombok and Alas total integrated transports, respectively. The correlation value between Alas and Lombok transport is 0.6, with a 99% confidence interval. The solid black line is sea level observed at the Benete station tide gauge. > High res figure
|
In general, there is good in-phase correlation (r = +0.6 with confidence interval 99%) between the throughflow in both the Lombok and Alas Straits, especially from December 2005 to April 2006. The northward flow in April 2006 is likely related to a Kelvin wave event (Sprintall et al., 2009). However, during the boreal summer, when strong seasonal upwelling occurred along the southern coast of the Nusa Tenggara island chain, no northward transport was observed in the Alas Strait (August and October 2006) as it was in the Lombok Strait. These reversal flows are also likely related to Kelvin wave events. Occasionally, Kelvin waves occur at depth and have no significant surface signature (Sprintall et al., 2009).
Similar to the Lombok Strait, Alas Strait transport is surface intensified, with the maximum velocity occurring at ~25 m depth. Due to frequent fishing and shipping activities in the Alas Strait, placement of our temperature sensors is limited to 30 m below the surface. To estimate the heat transport of the Alas flows, we used CTD data routinely collected by PT AMNT (nearly every month from November 1999 to November 2007) from the eastern side of the Lombok Strait (near Benete Bay) to fill the top 30 m of the temperature profile. Based on the temperature profile’s monthly climatology mean, a difference between the CTD and the mooring temperatures ranging from 0.5°C to 1.8°C causes error and uncertainty in calculating the heat flux. The heat flux is estimated based on the transport-weighted temperature or the throughflow’s effective temperature. Thus, the vertical distribution of transport and temperature controls the heat flux carried by the ITF flow in the Alas Strait. The mean transport-weighted temperature of the Lombok Strait from 2004 to 2006 is 21.5°C (Sprintall et al., 2009). Because of its shallower sill compared to that of the Lombok Strait, the Alas Strait carries the warmer ITF (25.7 ± 1.8°C). While allowing for vertical stratification in each layer across the strait, the errors and uncertainty of transport-weighted temperature may increase due to the assumption that there is no horizontal gradient in temperature and current across the strait.
Discussion and Conclusions
Fluxes of heat and mass in the Indonesian Throughflow measured over the last three decades demonstrate that it plays a significant role in the global ocean thermohaline circulation. While the measurements have been concentrated in the main inflow passages (the Makassar Strait and the Lifamatola passage) and the outflow passages (the Lombok and Ombai Straits and the Timor passage), the outflow straits along the Nusa Tenggara island chain (i.e., the Alas and Sape Straits) have been neglected. Now, for the first time, we have direct measurements of velocity and temperature collected at the Alas Strait. Our measurements partially overlap those from the International Nusantara Stratification and Transport (INSTANT) program (Sprintall et al., 2004, 2009), allowing us to compare velocity and temperature profiles, total volume integrated transport, and transport-weighted temperature. Our measurements reveal the complex ocean dynamics of this fascinating region, which depend on local and remote forcing and encompass many timescales.
There is a strong correlation between current velocity and throughflow in the Alas and Lombok Straits. Both are strongly affected by the regional Asia-Australia monsoon, and modulated by intraseasonal and interannual variability associated with Kelvin waves, ENSO, and IOD. Southward transport toward the Indian Ocean is more robust during the boreal summer (southeast monsoon from April to October) than during the boreal winter (northwest monsoon from October to April). The direct measurements coincided with the 2006 El Niño and a positive IOD, which prolonged the anomalously easterly winds until December 2006 and induced strong upwelling, cooler temperatures, and lower sea level at the southern coasts of the Nusa Tenggara island chain. Consequently, these two events generated stronger throughflow during the extended southeast monsoon.
There is interannual variability in the phase delay between the throughflow observed in the Makassar Strait and the Lombok and Alas Straits. During the 2006 El Niño and the positive IOD, the annual mean ITF transport in the Makassar Strait was lower than in a normal or La Niña year (Gordon et al., 1999, 2010, 2019; Ffield et al., 2000; Susanto et al., 2012; Sprintall et al., 2014). In contrast, the Alas and Lombok Straits (as well as the Ombai Strait and the Timor passage; Sprintall et al., 2009) exhibited higher transport during the El Niño year and the positive IOD. One plausible explanation for these observations relies on the strength of remote forcing associated with ENSO and the IOD and the divergence/convergence of the Banda Sea (Gordon and Susanto, 2001). More extended time series are needed to resolve the competing remote influences of ENSO and the IOD on the ITF transport variability during 2006. Longer time-series measurements should be collected within the straits along the Nusa Tenggara island chain, in addition to the Lombok and Ombai Straits and the Timor passage, to create a complete picture of the ITF and associated heat and freshwater fluxes into the Indian Ocean.
“…the Alas Strait transport serves a double role in the total ITF transport into the Indian Ocean: it enhances the total ITF during the boreal summer and reduces the total ITF during the boreal winter.”
|
The intraseasonal to semiannual signals that are associated with coastally trapped Kelvin waves generated in the equatorial Indian Ocean and that propagate along the southern coasts of Sumatra-Java-Nusa Tenggara require further investigation. Direct mooring observations indicate the existence of Kelvin waves in the Sunda Strait (Susanto et al., 2013; Li et al., 2018; Xu et al., 2018), the Lombok and Makassar Straits (Sprintall et al., 2000; Susanto and Gordon, 2005; Drushka et al., 2010; Susanto et al., 2012), and the Ombai Strait and the Timor passage (Sprintall et al., 2009). The northward reversal of flow/transport in the Lombok and Alas Straits in April 2006 is likely related to the Kelvin wave event. Similarly, the current reversal from southward to northward in August and October 2006 in the Lombok Strait may be associated with Kelvin wave events. However, these events were not observed in the Alas Strait. Therefore, in addition to a strait’s geometry (width and depth), the dynamics and characteristics of the Kelvin waves control whether they can enter shallow straits such as Sunda and Alas. Northward reversal flows during the intrusion of Kelvin waves reduce the ITF into the Indian Ocean and at the same time carry Indian Ocean water into the internal Indonesian seas. Further studies are needed to understand the dynamics of these Kelvin waves and are essential to more accurately estimating the ITF.
In addition, given that the Alas Strait is located next to the Lombok Strait and that they show some amplitude and phase variabilities in velocity, future simultaneous field measurements of the ITF should include all exit passages into the Indian Ocean (the Sunda, Bali, Lombok, Alas, Sape, and Ombai Straits, and the Timor passage) to avoid redundancy in estimating ITF and associated heat and freshwater fluxes into the Indian Ocean. For example, waters from the Indian Ocean entering the internal Indonesian seas during boreal winter (i.e., via the Sunda, Lombok, and Alas Straits) and re-exiting into the Ombai Strait and the Timor passage have been counted as ITF. Detailed numerical modeling studies are needed to resolve the total ITF. The inflow transport from the Indian Ocean to internal Indonesian seas likely contributes to the imbalance between the inflow and the outflow of the ITF (Gordon et al., 2010).
Knowledge of the dynamics and circulation in the Alas Strait not only contributes to understanding the total ITF and associated heat flux into the Indian Ocean, which is important for global ocean circulation and climate and input for general circulation models, it is also of interest to Batu Hijau mining company management in placing the submarine tailing system. The current patterns south of Lombok and Sumbawa islands are of interest to the mining company because they influence the distribution of the water column tailing plume. Although the bulk of the tailings flow down the submarine canyon as a gravity flow before they finally settle at the base of the Lombok Basin, a small fraction of fine suspended material associated with the tailings will be transported with currents before settling on the seafloor. The persistent southward flows of lower layer ocean currents in the Alas Strait support the company’s decision to dispose of tailings in the ocean via a 3.2 km submarine pipeline whose exit is located at a depth of 125 m at the head of the very steep Senunu Canyon. From here, the tailings are expected to flow down the canyon’s axis to their final deposition location in the Lombok Basin at depths greater than 3,000 m. The results of our research in the Alas Strait serve as an example of how collecting and analyzing oceanographic data are essential to the management of environmental issues facing mining companies located near coasts.
Acknowledgments
We are grateful to the Amman Mineral Nusa Tenggara mining company’s management, which provided the current velocity, temperature, and wind data used in this study. R.D. Susanto is supported by NASA grants 80NSSC18K0777 and NNX17AE79A through the University of Maryland.