Introduction
The Deepwater Horizon (DWH) oil spill was the largest ever in United States (National Commission, 2011) and the only Spill of National Significance. However, large oil spills, defined by NOAA (2017) as exceeding 100,000 barrels (420,000 gal) of oil are relatively rare, and very large ones even more so. Between 1969 and 2017, only 44 large oil spills (45 including the long-leaking Taylor Energy wells in the Gulf of Mexico; Mason et al., 2019) have occurred in US waters (NOAA, 2017), with about one-third of these in the Gulf.
Although they are rare, large oil spills can adversely impact the health of responders, cleanup workers, and residents, and the public welfare of affected communities (Walker et al., in press; Figure 1). The DWH spill also resulted in deaths of 11 oil industry workers. Physical and mental health effects have been reported and are closely related in disaster contexts (Aguilera et al., 2010; Palinkas, 2015; Ohrnberger et al., 2017; H.J. Osofsky et al., 2018), in part because environmental contamination results in significant stress (Hallman and Wandersman, 1992). How people adapt to repeated exposure to stress depends on an array of psychological, sociodemographic, economic, social, physical, and other variables (McEwen, 2005; Norris et al., 2008). Inhabitants of the Gulf region are particularly susceptible to oil spill health impacts due to widespread, preexisting health disparities, continuing exposure to contaminants, and location in a disaster-prone region (Lichtveld et al., 2016; Slack et al., 2020). Not surprisingly, the DWH oil spill, like Hurricane Katrina and other disasters, “had its greatest impact among those with the least” (Abramson et al., 2010).
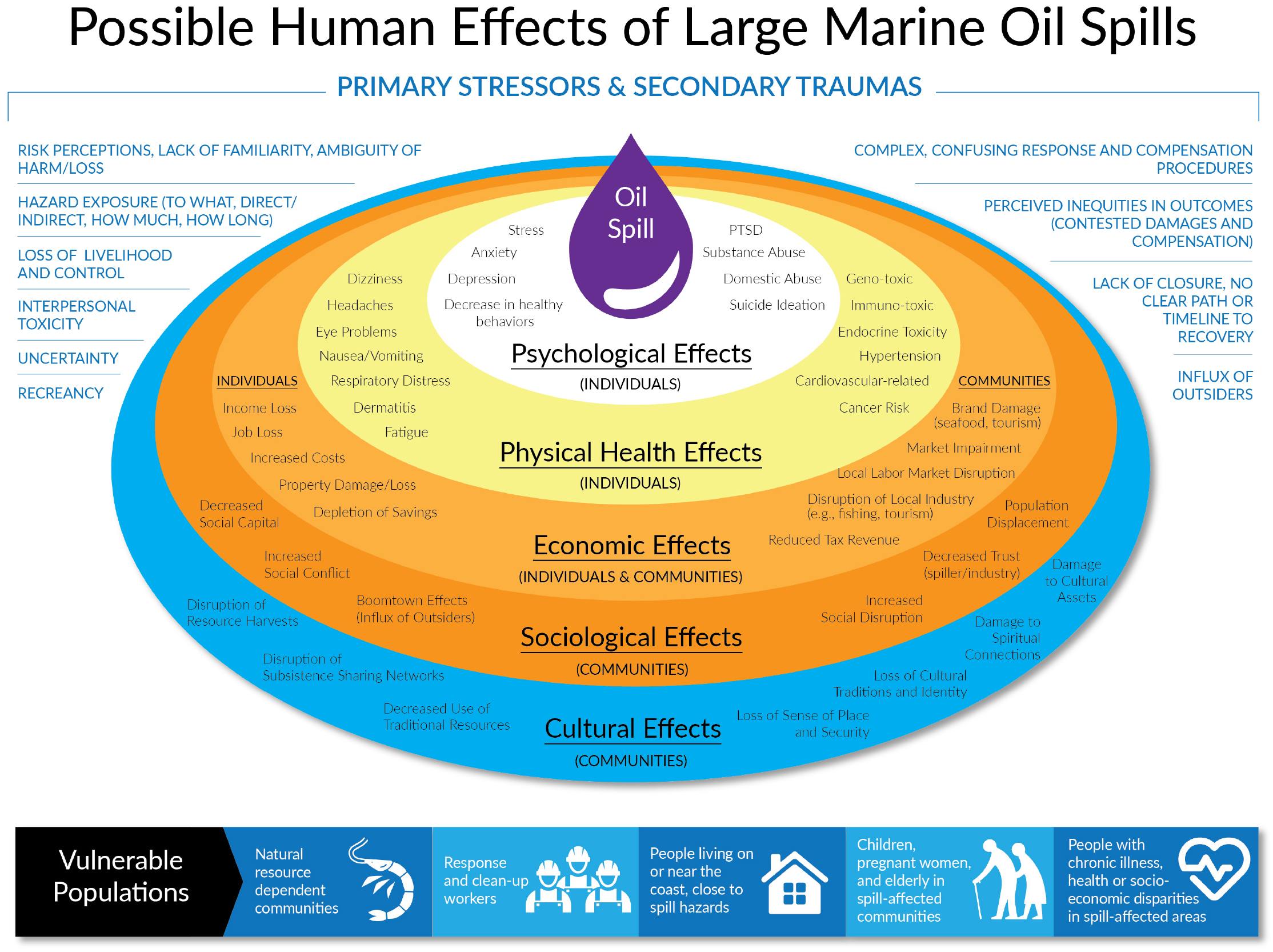
Figure 1. A summary of human effects research findings from studies on nine large oil spills: Exxon Valdez (USA), Braer (UK), Sea Empress (UK), Prestige (Spain), Tasman Spirit (Pakistan), Erika (France), Nadhodka (Japan), Hebei Spirit (South Korea), and Deepwater Horizon (USA). Relatively few spills have been shown to directly and adversely affect human mental and/or physical health, although many may have socioeconomic, ecological, or other effects of concern (Murphy et al., 2016). Whether any direct human effects occur from future spills will depend upon incident-specific conditions such as spilled oil type and volume, location, time of year, response actions, and safety and health protocols. Human susceptibilities to oil spill effects may be increased by pre-existing conditions, incident-specific and general life stressors, traumas, and previous disaster experience. Original concept developed by NASEM (2017) and adapted from Figure 3 in Beyer et al. (2016). Redrawn with permission from Sandifer and Walker (2018), with modifications. > High res figure
|
From its beginning, the Gulf of Mexico Research Initiative (GoMRI) recognized the need for human-focused as well as environmental impact research. It sponsored a public health workshop and broadly advertised a request for research proposals focused on human health effects. Although articles explicitly focused on human health comprised only a small portion of research publications supported by GoMRI funding, they present important findings (Eklund et al., 2019). Additional health-related research was supported via the US National Institute of Environmental Health Sciences (NIEHS), in part with BP funding, some of which is summarized here.
DWH impacts on individuals, families, groups, businesses, and communities were extensive, and they compounded negative effects of previous disasters such as Hurricane Katrina in the Gulf. In turn, these effects are likely to exacerbate traumas of subsequent disasters as well as ongoing threats from chemical pollution, oil seeps, and harmful algal blooms. In this article, we describe potential, perceived, and actual impacts, beginning with the people expected to be most exposed to toxic substances—the response and cleanup workers—followed by effects on those who resided in spill-affected areas, possible hazards to children at play on beaches, seafood contamination risks, and socioeconomic effects on the Gulf’s iconic fisheries and tourism industries and communities. We conclude with a discussion of the need for a human health observing system in the Gulf and a list of major findings and critical research needs for the future.
Health Effects on Responders and Clean-up Workers
Oil spill response and cleanup workers (hereafter referred to as workers) can be exposed to a variety of hazards, including the oil and its components, burning oil, dispersants, and cleaning agents, plus mixtures of oil, dispersants, and other chemicals. Other work stressors include high heat and humidity, musculoskeletal strain, long working hours, and financial (e.g., job loss) and psychological (e.g., depression, anxiety) effects.
Between 1970 and 2009, there were 458 tanker spills greater than 700 metric tonnes (approximately 222,500 gals) of oil (IOTPF, 2009); 38 of those spills affected human populations. Of those 38 spills, only eight that occurred between 1989 and 2007 were studied in some detail for human health effects (Kwok et al., 2017a): Exxon Valdez (US), Braer (UK), Sea Empress (UK), Prestige (Spain), Tasman Spirit (Pakistan), Erika (France), Nadhodka (Japan), and the Hebei Spirit (South Korea) (B.-M. Kim et al., 2009; Aguilera et al., 2010; Jung et al., 2013). Response to the Exxon Valdez oil spill led to many changes in oil spill preparedness, training, response, and worker safety and health, including use of personal protective equipment (PPE) in the United States (OSHA, 2020) and globally. Most studies of human health effects occurred in non-US countries where many workers from the community as well as volunteers engaged in oil spill cleanup with little protective gear. Studies that included pre-disaster health data were generally small, had shorter follow-up periods, and primarily investigated acute health symptoms. Acute health impacts were studied for up to one year after oil spill exposure in workers and affected community members to assess anxiety and post-traumatic stress, eye and skin irritation, and respiratory tract consequences (Palinkas et al., 1993; Zock et al., 2007; Na et al., 2012). Several studies reported longer-term respiratory symptoms in workers responding to the Hebei Spirit (Gwack et al., 2012) and Prestige (Zock et al., 2012) oil spills.
Human health studies following the DWH spill, the largest of their kind in history, are ongoing. Two epidemiological studies examine effects on the health of workers: the NIEHS Gulf Long Term Follow-Up Study (GuLF STUDY; Kwok et al., 2017a) and the US Coast Guard (USCG) Deepwater Horizon Oil Spill Cohort study (Rusiecki et al., 2018). These study teams have collaborated, and both are assessing potential long-term effects, including cancer.
The GuLF STUDY is assessing a range of human health effects, including worker access to mental health services (Lowe et al., 2015). It uses extensive data on actual and estimated exposures and health outcomes derived from surveys, home visits, and clinical records. Such limitations as self-reporting errors, confounding factors, and potential biases are documented in all papers and are frequently investigated with sensitivity analyses. The full cohort includes 32,608 people. Approximately 25,000 of these were actual workers, and the remainder were non-workers for comparison (i.e., people who were trained but not hired). The period of oil spill work activities was from April 20, 2010, through June 2011. Workers performed a variety of tasks with different exposure profiles under the primary categories of response, support operations, cleanup on water, decontamination, cleanup on land, and administration (Kwok et al., 2017a). The ultimate goal of the GuLF STUDY was to quantify exposures to total hydrocarbons (THC) and BTEX-H (benzene, toluene, ethylbenzene, xylene, hexane) via air measurements as a means of estimating potential toxic effects of THC and BTEX-H on workers (Kwok et al., 2017a).
The USCG cohort study consisted of 53,519 USCG personnel, 8,696 of whom responded to the spill and 44,823 who were not responders. The USCG study used health data from military medical encounters and cross-sectional survey data. Importantly, the USCG study can access a substantial amount of baseline health data for its participants, because medical data are available for all active-duty Coast Guard members from 2007 forward (Rusiecki et al., 2018). For the study, exposure levels and symptoms were based on self-reported and clinical data from the total cohort. While many health studies use self-reported information, including for toxic exposures, such data can be subject to recall and other biases (IOM, 1994; Williamson, 2007). Both the GuLF and USCG studies are continuing, with additional health effect papers expected.
Worker Exposures
To determine effects, physical health assessments evaluate elements of exposure pathways (ATSDR, 2005). THC and BTEX are generally considered to be the more toxic components of oil. THC is a composite of the volatile chemicals from the oil and was used as a surrogate for the workers’ “oil experience.” The exposure assessment used BP monitoring samples and agency short-term studies and accounted for oil changes due to weathering.
Kwok et al. (2017a), Rusiecki et al. (2018), and Ng et al. (2019) provided details of potential worker exposures and their measurements and estimations. Primary exposure pathways to the oil, burning oil (particulates), and oil spill chemicals are inhalation and direct contact (skin and eye). Due to the way offshore and onshore air quality measurements and exposure estimates were made, reported or observed symptoms cannot be linked to a specific crude oil chemical. From available data, it appears that total hydrocarbon exposure levels for workers likely were low compared to occupational standards (Middlebrook et al., 2012). However, an onshore study conducted from May 1 to September 30, 2010, assessed coastal ambient air quality for benzene and particulate concentrations (PM2.5; Nance et al., 2016) using air monitoring data from the US Environmental Protection Agency (EPA) and BP. The EPA’s Air Quality Index was compared prior to and during the spill. Onshore concentrations were generally higher following the spill, with benzene 2 to 19 times higher and PM2.5 10 to 45 times higher. Both concentrations were high enough to exceed public health criteria, with coastal areas near the spill and cleanup activities indicating measurable exposure disparities.
Worker Health Findings
GuLF STUDY findings to date have included nonfatal and fatal heart disease and reduced lung function in some workers. Reduced lung function has been documented in workers exposed to oily plants and wildlife compared to unexposed workers (Gam et al., 2018a). While Kwok et al. (2017b) linked reduced lung function with adverse mental health outcomes, Gam et al. (2018 a,b,c) found no association of depression and post-traumatic stress with lung function. Lawrence et al. (2020) reported improvements in lung function decrements four to six years after the spill, with those with the highest exposure exhibiting the greatest improvement.
When Strelitz et al. (2018) compared individual workers involved in DWH cleanup work with non-workers, they found a positive association between several oil spill related exposures and an increased risk of nonfatal heart attacks one to three years post spill. Factors associated with oil spill work, such as heat, strenuous conditions, physical exertion, and the health limitations of the individual worker, make it difficult to ascertain whether the risk of heart disease can be related directly to exposure to oil spill pollutants (Strelitz et al., 2019a). However, risk of heart disease has been associated with oil pollutants, cleanup activities, burned oil particulates, and volatile organic compounds (Strelitz et al., 2019b). The emotional stress related to the spill was also a possible cause of increased physical health risks such as heart attacks (Strelitz et al., 2018). Other analyses suggest that physical health symptoms contribute to cleanup workers’ risk for mental health issues (Lowe, 2016). However, fishers who had longer periods of cleanup work and thus potentially higher work-related oil exposure also had higher income, which in turn is associated with lower anxiety and depression (Lowe et al., 2016).
The USCG study reported positive associations between exposure to crude oil, and in some cases oil and dispersant mixtures, and acute respiratory symptoms (Alexander et al., 2018); neurological symptoms including headache, lightheadedness, difficulty concentrating, numbness/tingling sensation, blurred vision, and memory loss/confusion (Krishnamurthy et al., 2019); heat stress (Erickson et al., 2018); and skin issues, as well as some gastrointestinal and genitourinary symptoms that have not previously been well evaluated (Rusiecki et al., 2018).
Dispersants
Dispersants warrant separate mention given the widespread public concern about their unprecedented use during DWH (Starbird et al., 2015; Kwok et al., 2017a). Although studied for decades by oil spill scientists, dispersants remain a controversial response option (Bostrom et al., 2015; Walker et al., 2018). Robust protocols for their application on the water surface were implemented during DWH (Houma ICP, 2010; ASTM, 2018), including monitoring for environmental effects and some air monitoring for 2-butoxyethanol, one of the components in Corexit™ EC9527A. Although 2-butoxyethanol had been removed from Corexit™ EC9500A as a result of health concerns related to the Exxon Valdez spill (NRC, 2005), it remained in Corexit™ EC9527A, which was used only in surface applications for about a month during the DWH spill (McGowan et al., 2017). Dispersants were also applied at the wellhead to mitigate impacts from surface oil slicks and to reduce levels of volatile organic compounds near the source. Because the measurements and support documentation related to the dispersants were insufficient to allow reliable estimation of exposure levels across the Gulf (Stewart et al., 2018), the GuLF STUDY used responses to survey questions to assess dispersant exposure and estimated that about 10% of its cohort could have been exposed to dispersants (Kwok et al., 2017a). Findings by McGowan et al. (2017) suggest associations between exposure to dispersants, specifically Corexit™ EC9527A or Corexit™ EC9500A, and acute adverse health effects (e.g., respiratory and eye irritation and chest tightness at the time of the oil spill work as well as symptoms that were present at the time of study enrollment one to three years after the spill). Although only 5% of the Coast Guard personnel who responded to the Deepwater Horizon Oil Spill Cohort study health questionnaire reported contact with both oil and dispersants, for example, through assignments to conduct Special Monitoring of Applied Oil Spill Technologies (Levine et al., 2011), self-reported adverse neurological health effects were worse for those workers than for those exposed only to oil, and heat exposure also exacerbated symptoms (Krishnamurthy et al., 2019). However, these authors cautioned that self-reported information can be subject to bias, and they did not have information about who was where and when in reference to possible exposures.
Health Effects on Non-Workers
Human impacts of oil spills are much less studied than environmental impacts (Murphy et al., 2016), physical health effects are better researched among spill workers than in other populations (Laffon et al., 2016; Eklund et al., 2019), and mental health distress is better researched among community residents (Finucane et al., 2020a). Many, if not all, of the health effects noted for workers probably also apply to the general population that may be exposed, although the magnitude of the exposures may be considerably greater for workers.
Oil and associated chemical components have a wide range of known or putative toxic outcomes, including endocrine disrupting, carcinogenic, cytotoxic, immunotoxic, mutagenic, and genotoxic effects (Solomon and Janssen, 2010; Bhattacharya et al., 2016; Du et al., 2016; Laffon et al., 2016; Doctors for Environment Australia, 2019; Holme et al., 2019). Exposures can occur through physical contact with contaminants in air, water, or on materials; disruptions of routine behaviors; socioeconomic impacts; or other pathways (Hobfoll, 1991; Eisenberg and McKone, 1998; Slack et al., 2020).
Physical health problems or indicators identified with oil exposure include assorted respiratory issues; irritation of skin, eyes, nose, throat; chest pain; cardiovascular disease; gastrointestinal complaints; headaches, dizziness, fatigue, memory issues; and abnormal blood cell counts and liver and kidney function tests (e.g., Nance et al., 2016; Singleton et al., 2016; Afshar-Mohajer et al., 2018, 2019; Strelitz et al., 2019a). Laboratory experiments suggest that dispersant and dispersant-oil mixtures produce effects indicative of lung diseases such as asthma and chronic obstructive pulmonary disease (Liu et al., 2016) and mixtures may affect the gut microbiome (J.N. Kim et al., 2012).
Evidence for mental health distress associated with the DWH oil spill is mixed (Finucane et al., 2020a). Two large, population-based surveys in the Gulf Coast region suggest only modest or minimal changes in mental or behavioral health—at the aggregate level—before versus after the DWH spill (Gould et al., 2015). However, results across a range of other, more targeted studies indicate increased reports from individuals of symptoms consistent with depression, anxiety, and post-traumatic stress (Grattan et al., 2011; H.J. Osofsky et al., 2011, 2015; Buttke et al., 2012a,b; Gill et al. 2012, 2014; Morris et al., 2013; Drescher et al., 2014; Cherry et al., 2015; Fan et al., 2015; Aiena et al., 2016; Rung et al., 2016, Gaston et al., 2017; Kwok et al., 2017b). Substantial portions of coastal households (e.g., nearly 38% of an Alabama sample; Ritchie et al., 2018) were involved directly or indirectly in DWH-related claims, settlements, or litigation activity. Research on the compensation process suggests it was perceived by residents as random and lacking transparency, and resulted in additional psychological stress for individuals and corrosive effects on communities (Mayer et al, 2015; Ritchie et al., 2018; Halmo et al., 2019).
Even years after the spill, Gulf Coast residents report DWH-associated distress, but this may vary for different social groups, in part because of differing prior trauma, life disruption (especially income loss), or available support (Arata et al., 2000; Grattan et al., 2011; Morris et al., 2013, Cherry et al., 2015; Rung et al., 2016; Ayer et al., 2019; Ramchand et al., 2019; Bell et al., 2020; Parks et al., 2020). Higher levels of social support, sense of community, and perceived resiliency seem to be protective against spill-related stress. However, social support was not ameliorative for all groups, such as those with high attachment to damaged resources (e.g., fishing households; Parks et al., 2020) or nonreligious people living in highly religious areas (Drakeford et al., 2019). Ayer et al. (2019) reported that, after controlling for other traumatic experiences, individuals with higher levels of DWH exposure were not at greater risk for behavioral health problems, except for illness anxiety.
Fishers and Seafood Workers
Fishers and seafood workers are often members of tight-knit cultural communities (Picou and Gill, 1996; Marshall et al., 2007), and the cultural identity of the Gulf Coast region is intertwined with fishing (Henry and Bankston, 2002). Short-term fishing moratoria were enacted immediately following DWH (see Seafood Safety section), and even when the moratoria were lifted, uncertainty about the contamination of fishing grounds continued for fishers and seafood workers (Simon-Friedt et al., 2016). In addition to pre-existing economic pressures (Harrison, 2020), the ongoing disruption and stress from the DWH spill contributed to the unique vulnerability of these workers (Gill et al., 2012; Lee and Blanchard, 2012; Cope et al., 2013, 2016; Parks et al., 2018). While greater social support is typically helpful in bolstering mental health, it may operate differently among renewable resource communities (Freudenburg, 1992; Gill et al., 2014). Indeed, Parks et al. (2020) found that fishing households with greater social support were more susceptible to depressive symptoms six years after the DWH oil spill.
Cope et al. (2013) evaluated suites of self-reported mental and physical health issues via the Louisiana Community Oil Spill Survey, conducted in spill-affected parishes in June 2010 while the DWH oil spill was ongoing, and again in October 2010 and April 2011. A physical health index was calculated based on responses to questions about how worries about the spill manifested as physical symptoms. The index was significantly higher (indicating more health concerns) among fishing households, and while the index declined in subsequent survey waves for those not involved in the fishing industry, it grew stronger over time for people in the industry. Parks et al. (2018) used the same data source to examine disruption of routine behaviors, including sleep, following the spill. On average, respondents reported difficulty with about one-third of the activities. Again, respondents with ties to the fishing industry were more likely than non-fishers to report disruption to routine behaviors.
Women, Pregnant Women, and Children
Results of previous studies revealing adverse reproductive health effects for people exposed to petroleum hydrocarbons led to concerns about potential impacts on pregnant women in the Gulf following the DWH spill (Merhi, 2010). In the case of the DWH event, women physically exposed to the spill or who experienced negative economic impacts reported physical symptoms such as wheezing or irritated eyes and nose (Peres et al., 2016). Similarly, pregnant women who lived near the Hebei Spirit spill site in South Korea reported more eye irritation, headaches, and pain than those further away (B.-M. Kim et al., 2009). With regard to reproductive health, Harville et al. (2018) found little evidence of DWH spill exposure being associated with increased miscarriages or infertility in women from southeastern Louisiana, although spills in Nigeria have been linked with increased mortality rates among newborn children (Bruederle and Hodler, 2019). Among patients (predominantly African American women) seeking care at a Federally Qualified Health Center in an underserved area affected by DWH, post-traumatic stress disorder was associated with headaches, chest pains, dizziness, or trouble sleeping (Langhinrichsen-Rohling et al., 2017). However, where patients received post-disaster, integrated health services, perceptions of personal resilience increased and negative physical symptoms decreased (H.J. Osofsky et al., 2018).
Children are especially vulnerable to oil spills due to their physiology (high respiratory and metabolic rates, developing immune and hormonal systems, small stature), behavior (e.g., inquisitive play; Tipre et al., 2017; Slack et al., 2020), and poorly developed ability to estimate risk (Fischhoff et al., 2010) (see section on Beach Exposures). Children exposed to the DWH oil spill were twice as likely to have mental and physical health problems compared to those who were not exposed, and African American children and those from low-income households had higher prevalence of health effects (Abramson et al., 2010). Based on health status reports for children four, six, and eight years after the spill, general health and numbers of recent physical health problems (respiratory symptoms, eye and/or vision issues, skin problems, headaches, or unusual bleeding) were worse in households that experienced physical exposure to the spill or job/economic losses (Slack et al., 2020).
Physical Health Effects to Populations Following Beach Contamination: Children’s Exposures as an Example
Health-related exposures associated with oil spills can be estimated through quantitative risk assessment of health impacts. The risk assessment process addresses hazard identification (chemicals of concern and their environmental concentrations), exposure assessment (human activities that lead to exposure through ingestion, skin contact, or inhalation), and dose-response evaluation (amounts of the chemicals likely to enter and be absorbed in the body (Figure 2; NRC, 2009; Ferguson et al., 2020a). The effect is an estimate of risk typically provided in units of probability.
Hazard Assessment
The first step in quantifying risks is to estimate the concentrations of oil spill chemicals within human exposure zones such as beaches. Thousands of chemicals can be found in crude oils. They can be broadly categorized into hydrocarbon, non-
hydrocarbon, organometallic, and metallic compounds (Huba and Gardinali, 2016). Chemicals of concern in crude oil, those potentially toxic to humans, are the volatile aromatic hydrocarbons, BTEX-H, and the polycyclic aromatic hydrocarbons (PAHs) (ATSDR, 2005). Mitigation of oil spills can also include the use of dispersants, for which potential toxicities to humans are under debate (Ferguson et al., 2020a; NASEM, 2020; Quigg et al., 2021, in this issue); their use would need to be considered in risk assessments for future oil spills. Of the chemicals listed above, the PAHs and their degradation products have the most significant impacts at beaches because they weather slowly and are generally the most toxic (ATSDR, 2005). Also, toxicological profiles are not currently available for many oil chemicals and degradation products and for vulnerable populations for which information is very limited (e.g., pregnant women and children; Aeppli et al., 2012; White et al., 2016; Farrington, 2019).
Immediately after the DWH explosion, large-scale sampling efforts were initiated by the EPA, BP, and other organizations. Their measurements of oil spill chemicals were available within environmental matrices, including nearshore water and beach sediments, where beachgoers could be affected. Although these data provide snapshots of chemical concentrations, a more complete picture in time and space possibly could be obtained by using the data in oil spill models to predict oil spill chemical concentrations in nearshore environments. The General NOAA Operational Modeling Environment (GNOME) was used to help inform the Coast Guard-led response efforts about the areal extent of oil contamination. Initial efforts have been made to evaluate how well predictions from GNOME coincide with chemical concentration measurements (Montas et al., 2020; J. Xia et al., 2020, 2021), opening the possibility for future use of operational models to forecast oil spill chemical concentration distributions and provide an important first step for risk assessment.
Exposure Assessment
Different groups of humans can be exposed to oil spill chemicals at beaches, including response workers who actively engage in cleanup of contaminated shorelines and recreational users of these areas. Here, we focus on the recreational user category, and specifically on children who may be exposed to incidental residual oil that remains after beach cleaning and also to low level oiling that may occur from continuous leaks (e.g., Taylor Energy wells, seeps) and the redistribution of sunken oil. As noted elsewhere, children are a vulnerable constituency for exposures to contaminants in soil and sands, including potentially at beaches (Freeman et al., 2005; Xue et al., 2007; Beamer et al., 2012; Ferguson et al., 2019). In addition to factors noted previously, a child’s mouth and nose are generally closer to the ground than an adult’s, resulting in greater inhalation of pollutants that accumulate in sediments. Also, children’s play habits involve intimate contact with and potential ingestion of beach sand (Shoaf et al., 2005), and they tend to dig, bury themselves, and sit in the very shallow water, which typically has the highest levels of contaminants (Shah et al., 2011; Wright et al., 2011). Although these behaviors are specific to the beach environment, they had not been quantified prior to research supported by GoMRI following the DWH. GoMRI-supported studies have quantified children’s behaviors on beaches in Miami, Florida, and Galveston, Texas, through surveys (Ferguson et al., 2019), sand adherence studies (Ferguson et al., 2020b,c; Perone et al., 2020; Tomenchok et al., 2020), and videotaping of children and translating the videos to quantitative values that describe child beach play behavior (Alicia Ferguson, North Carolina Agricultural and Technical State University, pers. comm., 2021). Collectively, these studies have documented child beach play behaviors that can now be utilized in risk assessment analyses.
Dose-Response Assessment
Effects from the uptake of chemicals are typically separated into two types: non-cancerous (acute and chronic) and cancerous. Non-cancerous effects are evaluated by comparing the estimated dose to minimum risk levels for chemical compounds. If the dose is greater than the corresponding minimum risk level, then non-cancerous illnesses are likely to occur. To estimate the probability of cancer, a slope factor is needed and can be obtained for some oil chemicals from toxicological profiles available through the US Center for Disease Control’s (CDC’s) Agency for Toxic Substances & Disease Registry and EPA’s Integrated Risk Information System.
Risk Assessment Framework
Available information about hazard characterization, exposure assessment, and dose-response assessment has been incorporated in a risk assessment framework. Although work is currently ongoing to quantify children’s activities, results to date indicate that children who play at beaches that were cleaned after oil contamination are unlikely to experience non-cancerous acute or chronic health effects. Additionally, increased cancer risks are very low, within the 10–6 order of magnitude (i.e., about one in a million; Black et al., 2016, Altomare et al., 2021), given toxicological information available at the time. Also, simultaneous exposure to multiple chemical contaminants was not compounded in the analysis. Moreover, mental health outcomes can manifest in physical outcomes from chemical exposures and were not integrated into the analysis.
Seafood Safety
Oil Spills and Fisheries Closures
Following all oil spills in marine waters, the potential contamination of seafood supplies and the subsequent risks posed to human health from seafood consumption are of major concern to government authorities and community members where the spill occurred (Gohlke et al., 2011; Dickey and Huettel, 2016; Wickliffe et al., 2018). The magnitude and duration of the DWH spill posed a significant threat to the well-being of Gulf Coast communities, many of which depend upon safe and productive fisheries (Dickey and Huettel, 2016). At the time of the spill, Gulf fisheries accounted for around 16% of all US domestic landings (Shepard et al., 2013), fueling concerns of Gulf residents and non-residents about the safety of the nation’s seafood supply.
In anticipation of potential contamination, surveillance testing of seafood around the periphery of the spill began on April 28, 2010. Just four days later, May 2, 2010, the first emergency fishery closure was announced. This was only the second time in US history that a fishery in federal waters was closed because of an oil spill (Fitzgerald and Gohlke, 2014). Shortly thereafter, on May 24, the federal government declared a fisheries disaster for the states of Alabama, Mississippi, Louisiana, and Florida (K. Xia et al., 2012). By early June, at the height of the spill, nearly 37% of federal waters in the Gulf of Mexico Exclusive Economic Zone were closed to fishing along with state waters extending from Louisiana to the panhandle of Florida and some public beaches (Ylitalo et al., 2012). Though federal fishing waters began to reopen as early as the end of June 2010, it was not until April 2011 that all federal waters, including those immediately surrounding the wellhead, were fully opened. Sampling of previously closed waters continued through June 2011 (Ylitalo et al. 2012). Although most fishery closures in state waters were lifted by August/September 2010, it was several more years before the most heavily impacted coastal waters in Louisiana were considered safe for fishing (Deepwater Horizon Natural Resource Damage Assessment Trustees, 2016).
Federal Response to Human Health Concerns
Higher molecular weight PAHs, like those that made up ~3.9% of the oil from the DWH well, are of particular concern for seafood safety because of their persistence in the environment, potential for uptake in aquatic species, and toxic or carcinogenic effects (Goldstein et al., 2011; Allan et al., 2012). Though the use of dispersants (Corexit 9500A and 9527) had been approved by the EPA, and they were determined to have minimal toxicity in laboratory experiments (Judson et al., 2010), their unprecedented use led to public concern about potential accumulation in seafood supplies (Ylitalo et al., 2012).
The federal response to seafood safety followed a similar approach to that used in the response to the Exxon Valdez spill in 1990, with minor modifications based on the difference in the nature of the Gulf oil and the environmental conditions in which the spill occurred (Ylitalo et al., 2012; Dickey and Huettel, 2016). Finfish and shellfish samples were collected from multiple seafood sources and at multiple locations and depths in areas free or cleared of oil (Ylitalo et al., 2012). Seafood samples were not collected from areas where oil was visibly present on the surface or chemically detected in the water column since these locations were closed to fishing (USFDA, 2010). Samples were screened for the presence and concentrations of 13 PAHs determined to have the most potential to harm consumers as well as dioctyl sodium sulfosuccinate (DOSS), an indicator compound for the dispersant Corexit 9500A. Levels of concern for each targeted compound were calculated based on a variety of factors that included estimated dose, mean adult body weight, and averaged seafood consumption rates for the 90th percentile of seafood consumers (USFDA, 2010). These levels were considered to be safe or associated with negligible cancer and non-cancer risk for the US population (Ylitalo et al., 2012). However, the risk level, estimated duration of exposure, and the use of national demographic values for body weight, consumption rate, and longevity were extensively scrutinized (Gohlke et al., 2011; Ylitalo et al., 2012; Wilson et al., 2015; Dickey and Huettel, 2016). There was particularly strong criticism around the adequacy of information about exposure risks and how those risks were communicated to vulnerable populations (Rotkin-Ellman et al., 2012; K. Xia et al., 2012; Sathiakumar et al., 2017), such as pregnant women and Vietnamese-American fishers and their families.
After nearly 10,000 samples and months of testing, federal and state authorities determined that Gulf seafood from reopened areas was safe for consumption (Ylitalo et al., 2012; Dickey and Huettel, 2016). While individual PAHs of the 13 considered to be most harmful and/or carcinogenic were detected in many seafood samples, the concentrations were at least two orders of magnitude below levels of public health concern (Ylitalo et al., 2012). Further, DOSS was detected in less than 1% of samples and at very low concentrations. Though heavy metals were also a concern for many, particularly for vulnerable populations (Zilversmit et al., 2017), they were not included in the federal or state testing protocols (Fitzgerald and Gohlke, 2014). However, trace metal levels in both finfish and shellfish samples did not exceed background levels as determined by independent testing (Fitzgerald and Gohlke, 2014; Zilversmit et al., 2017).
Sathiakumar et al. (2017) specifically examined additional exposure risks to children from the coastal area of Mobile, Alabama, compared to children living inland. They concluded that there was no increased risk of exposure despite higher seafood consumption rates among coastal children compared to the general population. Further, Wickliffe et al. (2018) found that, even with consumption rates of both shrimp and finfish set at highly conservative values for human health (0.7 lbs [0.3 kg] per week and upward of 8.3 lbs [3.8 kg] per week, respectively), nearly three times the rates used to calculate the federal threshold levels of concern, concentrations in seafood samples did not constitute an unacceptable lifetime cancer risk.
Widespread fisheries closures following the DWH event reduced the probability of contaminated seafood entering into commerce. However, comparisons of pre- and post-spill consumption of seafood in multiple populations found a significant decrease in consumption in the immediate aftermath of the spill (Sathiakumar et al., 2017; Zilversmit et al., 2017; Wickliffe et al., 2018). Though the federal response to the spill, including the seafood testing program, drew much public scrutiny and concern, results from independent studies largely corroborated the federal effort. While some researchers found shortcomings in the federal approach, including the narrow scope of the PAHs included in chemical screening (Andersson and Achten, 2015; Dickey and Huettel, 2016; Farrington, 2019), based on toxicity information available at the time, no studies concluded that there was an excess exposure risk from consuming Gulf seafood in the months and years after the oil spill (Gohlke et al., 2011; Dickey and Huettel, 2016; Wickliffe et al., 2018). However, it is also largely agreed that the communication of exposure risks in general, and to vulnerable populations specifically, left much to be desired (Greiner et al., 2013; Dickey and Huettel, 2016).
Socioeconomic Impacts on Fisheries, Tourism, and Communities
The DWH spill resulted in numerous socioeconomic impacts, including effects on the fishing, tourism, and transportation sectors, and others that may affect community vulnerability and resilience. Similar to investigations related to human health impacts, GoMRI supported research on DWH socioeconomic effects but at comparatively modest levels, with resulting publications constituting only approximately 6% of total GoMRI-funded publications and about 4.2% of total competitive grant funds awarded (Petrolia, 2014; Finucane et al., 2020a). While much progress has been made in longitudinal monitoring of biophysical parameters, there is a lack of similarly robust efforts to monitor a suite of socioeconomic variables that could be used to assess the value of non-market resources, or identify cultural attributes, attitudes, social connectivity, or resilience in an oil spill or other disaster context (NRC, 2013; Yoskowitz et al., 2015).
Economic Impacts
In an initial study, Sumaila et al. (2012) utilized input-output analysis to estimate economic impacts for commercial fishing, recreational fishing, and marine aquaculture. They projected the highest total economic losses to commercial and recreational fishing, US $4.9 billion and $3.5 billion, respectively. The largest commercial fisher losses were predicted for shrimpers, which accounted for almost 85% of the study’s projected impact to that sector. In a separate analysis, Asche et al. (2012) suggested that, due to market integration, any increase in prices (driven by spill-related reductions in supply) would not offset expected revenue losses to domestic shrimpers. Rather, imports of farmed shrimp would rapidly increase to satisfy demand, meaning that while consumers would be mostly unaffected, producers could face significant and additive supply shocks. Overall, such impacts were expected to result in significant loss of income to shrimpers, as well as in boat building or repair, restaurants, and other businesses.
Notably, analysis is still needed to confirm economic impacts to fisheries 10 years after the spill. For example, while studies alluded to significant impacts to US shrimpers, in 2011, the year following the DWH spill, the Gulf produced >216 million pounds (98 million kilograms) of heads-on shrimp at a commercial landings value of >US $421 million (NOAA, 2020), accounting for about 68% of US shrimp landings that year (NRC, 2013). For comparison, average Gulf shrimp landings for the period 1990–2009 were 236 million pounds (107 million kilograms), with a range of 181–290 million pounds/yr (82–132 million kilograms/yr). While evidence does show reduced demand and a decrease in seafood sales directly after the spill (McGill, 2011), it is not clear whether these effects persisted. For example, the total commercial landings value for all species in the Gulf (Texas, Louisiana, Mississippi, Alabama, and Florida West Coast) for the seven years following the spill (2011–2017) was US $6.8 billion (Consumer Price Index [CPI] adjusted), whereas landings for the seven years preceding the spill (2003–2009) had a total CPI adjusted value of US $5.9 billion (NOAA Fisheries data, CPI adjusted). Using biophysical projections from the Atlantis ecosystem model (Ainsworth et al., 2018) with input-output analysis, Court et al. (2020) modeled total economic impacts for the 10 years following the spill for commercial fishing revenues and recreational fishing expenditures. Results indicate that, across both sectors, impacts could include losses totaling US $2.3 billion composed of $1.2 billion in gross regional product, $700 million in labor income, and $160 million each in federal and state tax revenues. However, more research is needed to reconcile modeled outputs and actual landings data, particularly for sectors anticipated to incur significant impacts such as the shrimping industry.
With nearly 223,000 km2 of fishable waters and public beaches closed or showing oil spill advisories during the months-long spill event (Ylitalo et al., 2012), the tourism industry was also impacted. Misperception also contributed to tourism impacts, with one report demonstrating that the public was unaware of locations and extent of damage and believing Louisiana waterways to be closed when they remained open (MDRG, 2010). Of those surveyed, 44% believed the oil spill caused damages on par with Hurricane Katrina, and 29% of tourists canceled or postponed planned trips to Louisiana due to the spill (MDRG, 2010). Another study found that about US $147 million in tourism and recreation claims were paid between August 2010 and March 2012. While this amount only represented 2% of total claims, it spanned 23 different business types within the industry, including airlines, aquaria, entertainment, water sports, wildlife watching, and more. Claims for impacts to retail, sales, and services were about US $1.9 billion representing 31% of total claims, while those for food, beverage, and lodging amounted to US $1.6 billion and 26% of the total (Eastern Research Group, 2014). Analysis of claims across the Gulf further found significant impacts on resorts, charter fishing, and marinas/docks/ice houses, though hotels and restaurants were most impacted. Effects differed among the states, with Texas receiving a relatively small amount of paid claims, while individuals in Florida and Louisiana received US $340 million and $227 million, respectively, and businesses US $164 million and $88 million, respectively (Eastern Research Group, 2014). Though individuals and businesses clearly suffered economic losses, counties with over 1,000 employees in tourism experienced only temporary or no declines in tourism-related employment, followed by growth, though there were exceptions. For example, Hancock County, Mississippi, experienced a 7.7% decline in tourism employment compared to 2009 (Eastern Research Group, 2014).
An important finding is that consumer perception matters and is an important driver of economic impacts. A review of news articles and interviews found numerous inaccurate portrayals of conditions, with media sensationalism contributing to a perception that the entire Gulf coast was contaminated with oil (Eastern Research Group, 2014). To counter such misinformation in the future, Nelson et al. (2018) offer a spatially explicit approach for evaluating and visualizing risks to tourism or other economic sectors.
Other studies also lend insights for future research. In a case study of New Orleans, Porter (2011) argues that fishing and tourism should be viewed as a cluster of overlapping geographic space, knowledge, and impact, for example, with tourism concentrated on recreational fishing and the seafood culture. Such interdependence may limit opportunities for growth where risks of economic and environmental crisis persist, yet innovation could address some risks to external shocks. For example, a tourism subsector could focus on ecotourism and demonstrate how the city and ecosystem remained resilient to the DWH disaster, which could provide a new kind of growth (Porter, 2011). Researchers also investigated non-market impacts of the oil spill. Alvarez et al. (2014) used choice modeling to estimate compensation due to marine anglers from non-market value losses at a mean of US $585 million with a 95% confidence interval. Petrolia (2014) offers that perhaps the most prominent change for research resulting from the disaster was an expansion to an ecosystem services approach to damage assessment. The National Research Council (NRC, 2013) assessed oil spill impacts on the provisioning ecosystem service of seafood and fish-based products, as well as the cultural services provided through recreational fishing (among other case studies). Results suggested that, while fishery closures may have decreased landings in the immediate aftermath of the spill (McCrea-Strub et al., 2011; NRC, 2013), increased catch rates were later observed for a large group of regularly harvested species (Fodrie and Heck, 2011; NRC, 2013). However, impacts from the spill to fishery harvest may take years or even decades to materialize, particularly regarding cascading effects through marine food chains (NRC, 2013).
Community Resilience Impacts
Resilience is “the capacity of a system to absorb shocks and disturbance and still maintain function” (Berkes and Folke, 1998), or “the capacity of a social-ecological system to adapt to change through self-organization and learning” (Berkes et al., 2003). Finucane et al. (2020a) examined a set of adaptive capacities that may diminish impacts of an ecological disaster like the DWH, finding that although the spill resulted in differing economic impacts across fisheries, tourism, and oil and gas sectors with location, the aggregate impacts were primarily short term. However, at the household level, and particularly in poorer households, financial impacts were still being felt years later. Further, community well-being showed signals of distress related to the spill across multiple studies. Distress was expressed differently across different groups, with those tied to natural resources for their livelihoods exhibiting higher rates, including through an erosion of trust (Finucane et al., 2020a). Similarly, Cope et al. (2016) found levels of distrust across communities regarding information provided by both BP and the federal government, with trust in state government being somewhat stable. Mayer et al. (2015) reported that, although the compensation process helped to mitigate economic impacts, apparent confusion, lack of transparency, and perceived inequality in the process eroded trust and strained community relations. In addition to those reliant on natural resources, other groups identified as having higher vulnerability to the spill included populations that exhibit disadvantages related to rural environment, dependence, older age, and socioeconomic and/or educational disparities, as well as living in mobile homes (Cope and Slack, 2017), being of minority ethnicity (e.g., Vietnamese; Patel et al. 2018), or being female (Lightfoot et al., 2020). In a case study of Apalachicola, place, heritage, and moral identity were found not to be fixed community attributes but rather to provide individuals with varying resources, which in turn impact disaster recovery (Clarke and Mayer, 2017). This has important implications for institutional recovery frameworks—often crafted by external actors—in a precarious Gulf region where resilience may vary locally. For future spills and other disasters, more attention should be given to the researcher-community relationship, where cultural norms and trust are critical for successful engagement with local residents (Lesen et al., 2019; Finucane, 2020b).
Need for a Community Health Observing System
Environmental disasters of various kinds and magnitudes occur frequently in the Gulf region (NOAA, 2020; Sandifer et al., 2020; Smith, 2020), with one often following another and compounding the impacts of the previous incident. Examples include the 2010 DWH oil spill that followed the catastrophic effects of Hurricane Katrina in 2005, and the health effects of which were then compounded by subsequent disasters such as Hurricane Harvey in 2017, and others (J.D. Osofsky et al., 2014; Shultz et al., 2015; SAMHSA, 2018). Recurrent disasters take a heavy toll on human health in the region, where many people already suffer significant health and economic disparities (Lichtveld and Arosemena, 2014; Lichtveld et al., 2016). Climate change, land subsidence, and population and development pressures are all likely to increase the incidence and severity of environmental disasters in the Gulf region, along with their accompanying adverse effects on human health (Sandifer et al., 2020).
Previous studies of health effects of Gulf disasters, as well as other sections of this article, demonstrate the lack of, and critical need for, baseline health information with which to compare effects of future disasters (Sandifer et al., 2020). Ongoing health monitoring is essential to develop and maintain such a baseline and capture acute, chronic, and long-term health impacts, as well as secondary complicating events that occur in the intervals between major disasters. Recognizing the urgent need for a health observing system in the Gulf of Mexico region analogous to the kinds of atmospheric and oceanic observing systems well established for monitoring and predicting hurricanes and other extreme weather events, GoMRI commissioned a design study. The intent was to devise a framework that would provide for continuous collection of health information to ensure existence of adequate pre-, during, and post-disaster information for comparative purposes and to improve emergency planning and public health response. The resulting framework (Sandifer et al., 2020) builds upon and leverages existing ongoing national health surveys and includes new longitudinal cohort studies designed to elucidate long-term health trends and disaster-associated health effects in the five Gulf states. The system’s new cohort studies must continue indefinitely, be large enough to represent the risk-prone coastal areas and populations known to be particularly vulnerable to disasters, and include mental and physical health assessments and measures of stress. In addition to collecting and providing information on direct and indirect health impacts to individuals, the system should also incorporate community data. As far as we are aware, this is the only observing system designed for a disaster-prone area and focused explicitly on disaster-related health effects.
Components of the proposed Gulf of Mexico Community Health Observing System would include: three cross-sectional surveys, the National Health and Nutrition Examination Survey (NHANES; https://www.cdc.gov/nchs/nhanes/), the Behavioral Risk Factor Surveillance Survey (BRFSS; https://www.cdc.gov/brfss/), and the National Health Interview Survey (NHIS; https://www.cdc.gov/nchs/nhis/); a proposed new Augmented BRFSS survey for the Gulf states; the new National Institutes of Health (NIH) All of Us national longitudinal study (https://allofus.nih.gov/); and proposed new Large, Small, and Disaster-Specific Gulf of Mexico longitudinal cohort studies (Figure 3). The cohort studies are designed to build upon one another and are the unique and most important parts of the observing system. Another significant strength of the system is its ability to adapt rapidly as needs arise and new biomedical and other technologies are developed.
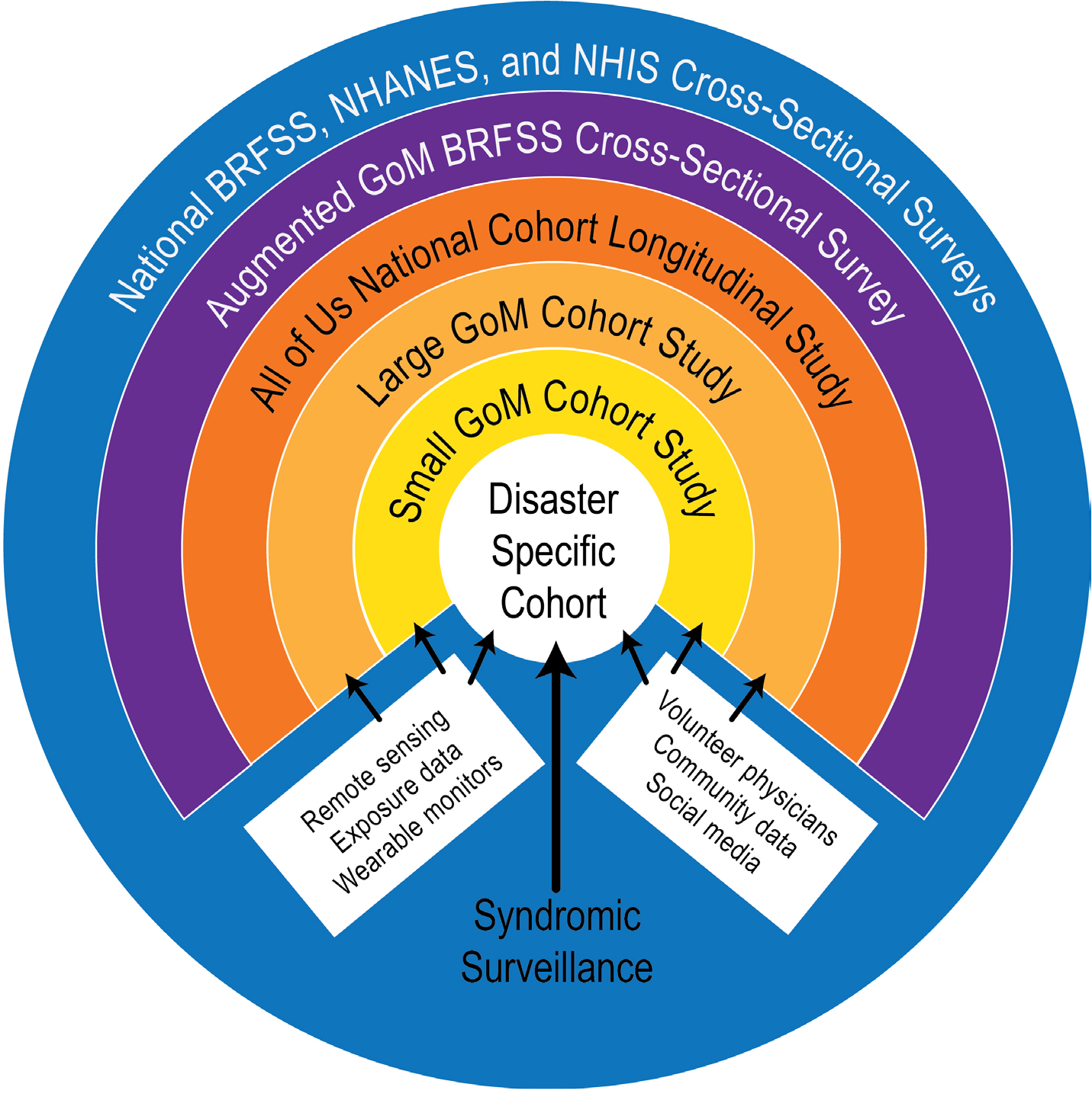
Figure 3. A conceptual framework for a Gulf of Mexico Community Health Observing System. From Sandifer et al. (2020). > High res figure
|
The geographic focus of the proposed observing system is the 68 coastal counties of the five Gulf states. These are counties that either have a Gulf shoreline or are near the coast and contain Federal Emergency Management Agency (FEMA)-identified areas with high risk for tidal and/or storm surge flooding (Ache et al., 2013). A statistically representative sample of volunteers from the populations in these counties is proposed, with stratification to ensure proportionate inclusion of both urban and rural populations and with additional, targeted recruitment as necessary to enroll adequate numbers of people deemed particularly vulnerable or typically underrepresented (e.g., ethnic minorities, the underserved, and those who suffer health, health care, and economic disparities). Initially, volunteer participants are expected to be recruited using a mail-address sampling frame, followed by use of electronic communication means to the greatest extent possible.
If implemented, assessments of mental and physical health in the new cohort studies will include information obtained via detailed health questionnaires, clinical examinations, biological specimens, electronic health records, and use of wearable health devices. These will be augmented with data from secondary sources such as information from State Health Departments and the CDC, national community surveys, environmental exposure databases, social media, remote sensing, and others. Biomarker data derived from biological samples are expected to be used as indicators of health status, including for calculation of measures of chronic stress and its impacts on physical and mental health.
Primary users of information from the health observing system will be public health and medical professionals, emergency managers and responders, and clinical and academic researchers. Secondary users are likely to include political, community, and business leaders, and many others. Data and information products are expected to be used to assess disaster-related health effects; enhance disaster planning and response; improve protection for disaster responders and workers; build individual and community resilience; and promote new clinical, biomedical, and public health research and practice.
Conclusions, Gaps, and Opportunities
A broad range of mental and physical health impacts has been attributed to oil spills in general and the DWH disaster in particular, but in most cases definitive cause-and-effect linkages are lacking. Overall, mental and physical health effects and their interactions are inadequately studied for oil spill workers, their families, and others who may be exposed to or affected by them. Besides spill workers, special attention is needed to vulnerable people, including individuals with chronic illness or who suffer health and health-care disparities and/or socioeconomic deprivations, lack strong social support, the elderly, and natural-
resource-dependent communities.
A common theme across studies is the overarching role of stress (e.g., from physical exposure, job or income loss, compensation/litigation processes, or behavioral disruption) as an important factor associated with poor mental and physical health outcomes.
Human health findings were severely limited by a lack of baseline health data, long delays in implementing major health research activities following the spill, heavy reliance on self-reported and cross-sectional survey data, limited collection of clinical health information, and a paucity of long-term, longitudinal cohort studies. Health studies need to be initiated before, during, or immediately following a large spill and must continue long enough to identify long-term effects and secondary surges of chronic illnesses. Critically needed are cohort studies that start before a major spill and continue through it and onward for a long period after.
Considerable information about components of oil (e.g., benzene) and their potential toxicity to humans was available before the DWH spill, but many gaps in knowledge were identified, including effects of field-relevant exposures to oil components, engine exhaust, and other chemicals (e.g., dispersants and decontamination cleaners) as well as these in combination with additional stressors such as heat and humidity. Recent studies indicate that children who played at beaches that were cleaned after oil contamination are at low risk for acute or chronic health effects. However, further development of models to forecast the distribution of fresh and weathered oil is needed to predict potential human exposures and inform the common response question, “How clean is clean enough?” Also, toxicological profiles are not currently available for many oil chemicals and degradation products and for vulnerable populations for which information is very limited (e.g., pregnant women and children). A better understanding is needed of impacts from exposures to multiple chemicals, and that incorporates mental health impacts from oil spills that contribute to adverse physical outcomes.
When fisheries were reopened following closures after the spill, Gulf seafood was demonstrated to be safe for human consumption within guidelines existing at the time. However, there was much uncertainty and persistent worry about seafood safety, even years after the spill. For future large spills that affect fishing zones, thorough and rapid appraisals of seafood safety should be undertaken immediately after the spills, followed by plain language communication regarding consumption risks based on appropriate demographic information (e.g., race/ethnicity, age, sex/gender identification, pregnancy, chronic illness, weight, seafood consumption habits), and there should be regular updates. Better health advisories targeted to vulnerable populations and those who use beaches and coastal recreation areas are needed. Collaboration between government and stakeholder groups for monitoring of seafood should be enhanced, and additional social science research should be supported to improve risk communication strategies and outcomes.
There is a lack of systematic collection and integration of socioeconomic data necessary to assess near- and long-term societal impacts of oil spills. Also lacking is a concerted effort to aggregate existing data, identify and fill gaps in longitudinal data collection, and make data and information products broadly available to enhance community disaster resiliency and recovery. As an example, even 10 years after the DWH spill, the long-term psychosocial impacts resulting from the extensive fisheries closures have yet to be fully understood, although extensive data have been collected.
Major Opportunities for the Future
Establishment of the proposed Gulf of Mexico Community Health Observing System would be a major step toward improving health care planning and response and in identifying and characterizing acute, chronic, and poorly known adverse health effects of oil spills and other disasters. The system could be modified for use in other disaster-prone regions.
To inform future oil spill response protocols, findings of worker-related health effects studies should be viewed in the context of oil spill response practices, including operationally relevant exposures, worker safety and health standards, and pollutant and dispersant monitoring protocols, perhaps facilitated by an expert workshop involving researchers, preparedness and response decision-makers, and the Occupational Safety and Health Administration (OSHA) (Holliday and Park, 1993; Science and Policy Associates, Inc., 1993).
There is an urgent need for and the opportunity to develop a socioeconomic observing system. Such a system would link the most significant available socioeconomic data streams, identify additional needed information and suggest how it should be collected, and aggregate the data so as to be useful in analytical efforts to accurately estimate social and economic impacts of future large spills.
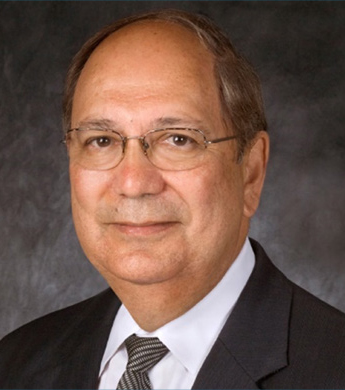 |
DEDICATION
This article is dedicated to the memory of Ciro V. Sumaya, MD, MPHTM, founding Dean of the Texas A&M School of Public Health and a member of the GoMRI Research Board. It was he who, in 2013, convened the GoMRI Public Health Workshop, the report from which has helped to guide GoMRI’s efforts ever since on the DWH explosion’s and spill’s effects on human health. We mourn his tragic death and miss him very much.
|
Acknowledgments
The authors thank Catherine Polk for the new rendition of Figure 1 and Landon Knapp for editorial and other assistance. We also appreciate the guidance provided by Burton Singer, Raymond Orbach, and Denis Weisenberg of the GoMRI Research Board, and helpful suggestions to improve the paper from two anonymous reviewers. This work was supported in part via contract #C-231826 between the Gulf of Mexico Alliance and the College of Charleston.
Author Contributions
P.A.S., lead author; A.F., M.L.F., M.P., H.M.S.-G., A.H.W., and K.W. contributed equally to the writing and were lead contributors for sections. R.C. and D.Y. contributed to the section on Socioeconomic Impacts.