Autonomous Acoustic Gliders for Area-Based Management
Area-based ocean management aims to balance human use of the marine environment with biological conservation (Maxwell et al., 2015). There are two primary management frameworks for achieving this: (1) static management areas (e.g., conventional marine protected areas) that are fixed in time (e.g., seasonally) and space based on historical data regarding the occurrence of species needing protection, and (2) dynamic management areas that are triggered in response to recent observations or predictions of species occurrence. Static management is typically applied to known critical habitats or where predictable aggregations of at-risk species frequently overlap with high-threat human activities (i.e., high risk areas). Alternatively, dynamic management is increasingly being used to address short-term, localized, changing, or ephemeral risks. This approach is applied in areas with irregular overlap of at-risk species with human activities, but where the impact of potential interaction is significant (i.e., high threat areas). Success of either framework relies on long-term, persistent biological monitoring using reliable ocean observation assets.
Electric gliders are mobile, cost-effective underwater surveillance tools that can be equipped with sensors for measuring oceanographic conditions and recording marine soundscapes (Webb et al., 2001). Glider deployments fill a unique whale surveillance niche compared to other standard platforms. Like visual surveys, gliders survey along transects, but their temporal effort is significantly higher, with deployments lasting up to six months during which monitoring is continuous, including at night and in all types of weather (Baumgartner et al., 2014, 2020). The mobility of gliders allows for regional-scale spatial surveys of habitats or management areas that span hundreds of kilometers and can be remote, a task not easily achievable with individual passive acoustic monitoring (PAM) moorings. Additionally, all profiling electric gliders carry a standard suite of oceanographic sensors for simultaneously monitoring cetacean acoustics and environmental conditions throughout the water column, which is not standard for PAM moorings or visual surveys (e.g., Ruckdeschel et al., 2020). Thus, gliders fill a unique surveillance role that is required to meet whale management objectives that rely on acoustic and environmental monitoring across seasons and variable spatial scales, including in near-real time.
Gliders equipped with PAM devices are capable of robust near-real-time monitoring of numerous whale species (Baumgartner et al., 2013, 2020). One such species is the North Atlantic right whale (Eubalaena glacialis, NARW), which is suffering an ongoing unusual mortality event that resulted in 151 documented mortality, serious injury, and morbidity cases from 2017 to 2024: 41 deaths, 39 serious injuries, and 71 sublethal injuries (note that only about one-third of right whale deaths are thought to be documented; NMFS, 2025). The coastal distribution of NARWs spans calving grounds in the southeastern United States to foraging grounds in northern United States and Canadian waters, resulting in frequent overlap with high density vessel traffic, major shipping lanes, and commercial fisheries operations. As a result, the leading causes of death and injury for NARWs are vessel strikes and fishing gear entanglements (Sharp et al., 2019). Unpredictable shifts have occurred in NARW distributions in recent years, likely linked to the consequences of climate change impacts on habitat suitability and feeding conditions (Meyer-Gutbrod et al., 2018). This resulted in changes to NARW co-occurrence with human activities as well as to existing protection measures. Therefore, glider effort is expanding over larger temporal and spatial scales to better understand and respond to the dynamic behavior of and persistent threats to this critically endangered species.
Glider-derived acoustic detections can provide information on the occurrence and distribution of NARWs in relation to high threat human activities at hourly to daily timescales. Many near-real-time PAM systems deployed to monitor NARWs (i.e., gliders and moored buoys) use a digital acoustic monitoring (DMON) instrument running a low-frequency detection and classification system (LFDCS; Johnson and Hurst, 2007; Baumgartner and Mussoline, 2011; Baumgartner et al., 2013, 2020) that automatically detects and classifies tonal baleen whale sounds in real time. A subset of these detection data is sent to shore periodically (e.g., when a glider surfaces), enabling acoustic analysts to validate detected whale calls in near-real time following a standard protocol (Figure 1; Wilder et al., 2023). Validated detections are then rapidly disseminated to stakeholders via various automated systems. Here, we highlight five case studies to discuss how DMON/LFDCS-equipped gliders are being used internationally for area-based monitoring of NARWs across habitats and in distinct environments, as well as how the acoustic observations are being used to inform management and/or stakeholder actions to mitigate the impacts of anthropogenic threats (Table 1). The goal of these examples is to illustrate how this platform’s unique surveillance niche can help address the complex and multifaceted management needs of a migratory endangered species.
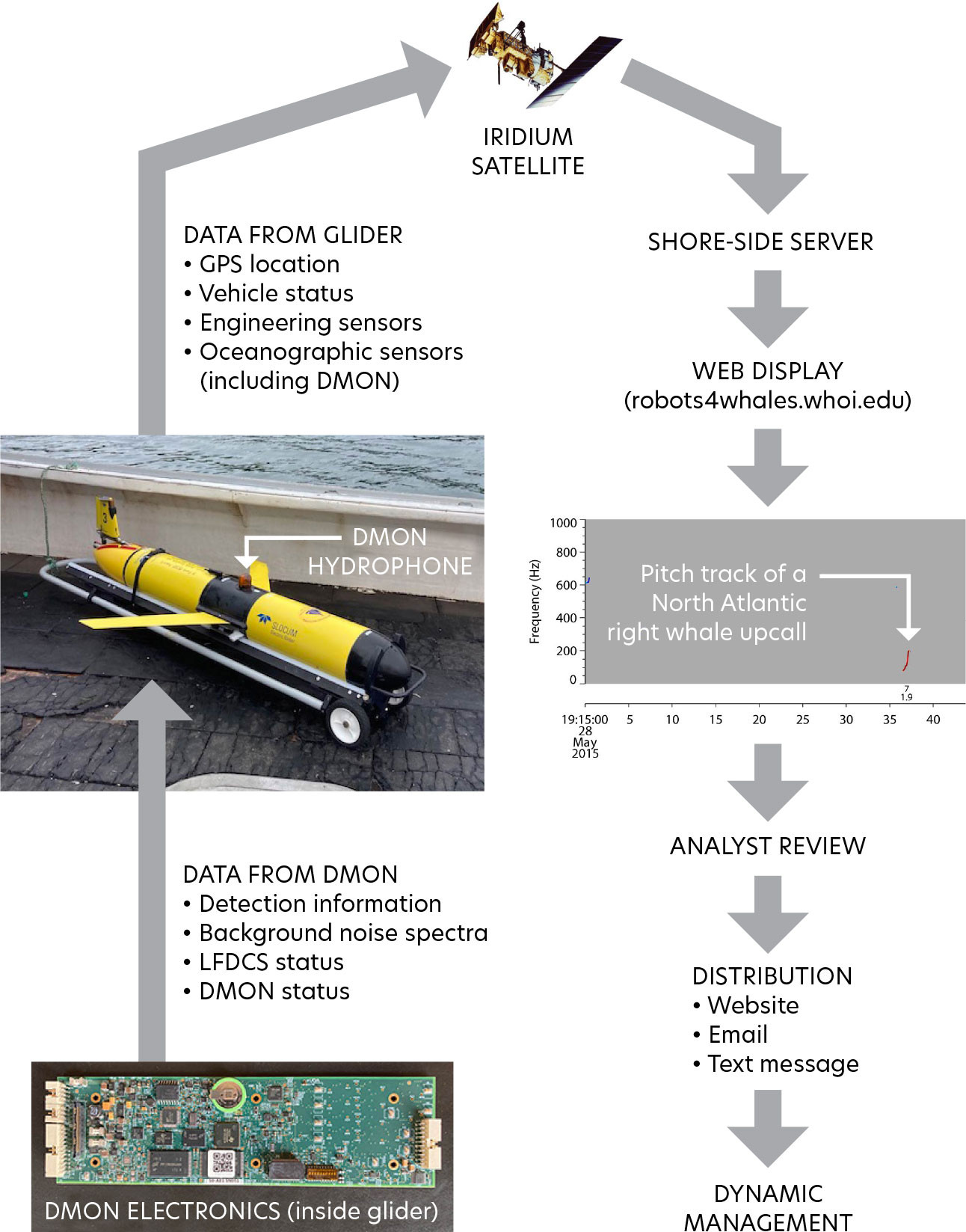
FIGURE 1. Diagram of whale acoustic detection data flow from a digital acoustic monitoring (DMON) instrument running a low-frequency detection and classification system (LFDCS) integrated into a Slocum glider to a shore-side server via the Iridium satellite service and displayed on a publicly accessible website. After analyst review, the presence of North Atlantic right whales is shared with stakeholders via the website and email/text messages. Depending on the area, dynamic management measures may be implemented in response to whale detections. > High res figure
|
TABLE 1. Summary of area-based monitoring of North Atlantic right whales (NARWs) across Canada and the United States, highlighting how glider-derived acoustic detections are being used to trigger management actions and/or inform stakeholder decisions to mitigate the impacts of various anthropogenic threats. > High res table
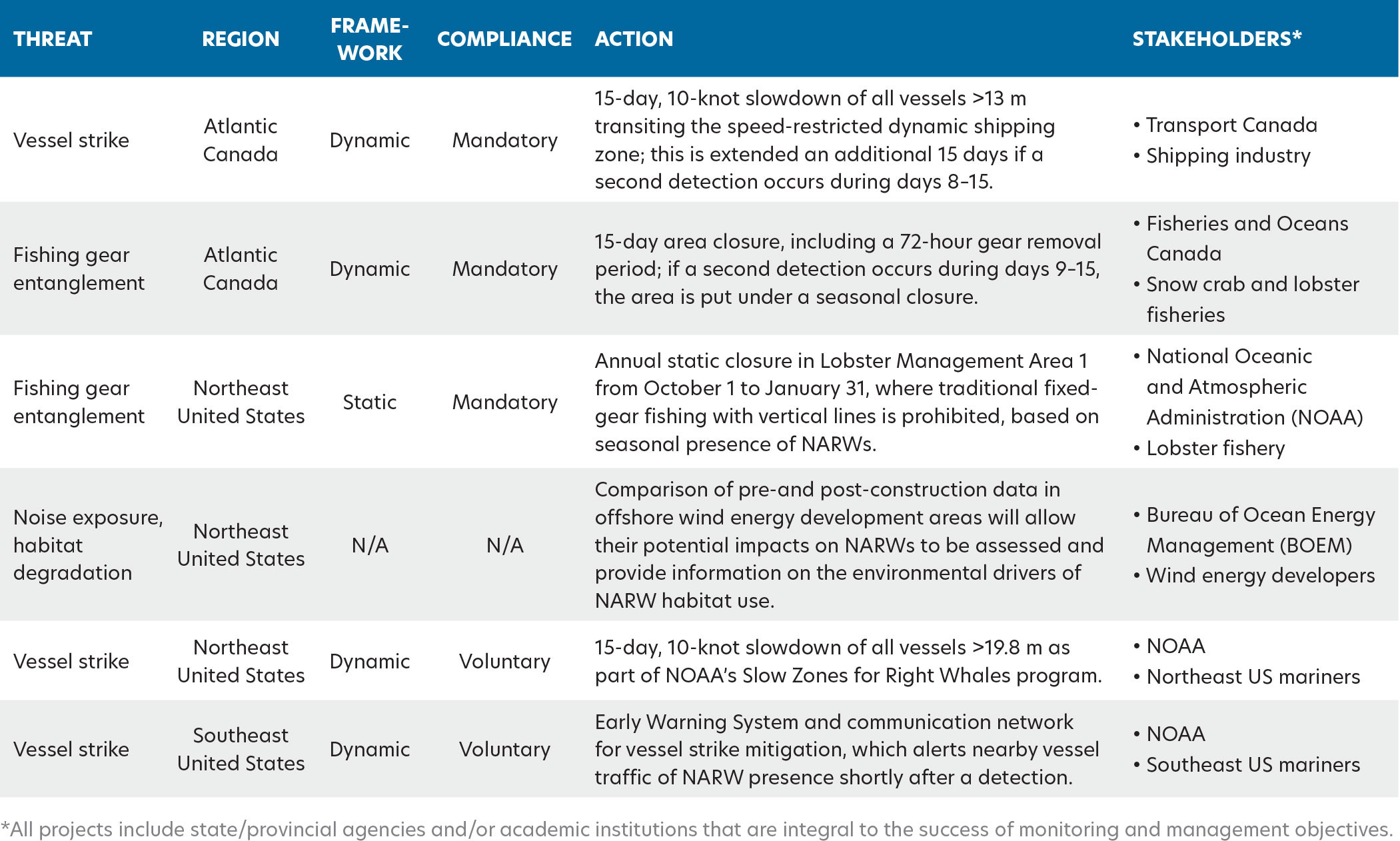
|
NARW Conservation Case Studies
Canadian Dynamic Shipping Zones
The Gulf of St. Lawrence (GoSL), Canada, recently became a foraging hotspot for NARWs (Meyer-Gutbrod et al., 2021). The GoSL is an inland sea bisected by shipping lanes that serve as the sole oceanic connection between North America’s Great Lakes (including Canada’s largest city, Toronto) and global ports. Regional overlap of whales and high vessel density has contributed to the species’ unusual mortality event (Daoust et al., 2018). To reduce the risk of vessel strikes to NARWs in this high threat area, the Canadian government developed a significant new dynamic management plan for shipping in 2018 (Transport Canada, 2024). Beginning in 2020, the plan included the implementation of PAM-equipped glider surveys within deep water (>300 m) dynamic shipping zones to trigger mandatory regional speed restrictions of 10 knots in response to NARW acoustic presence (Figure 2). These surveys are conducted annually from April to November and are done in collaboration with the University of New Brunswick and Dalhousie University.
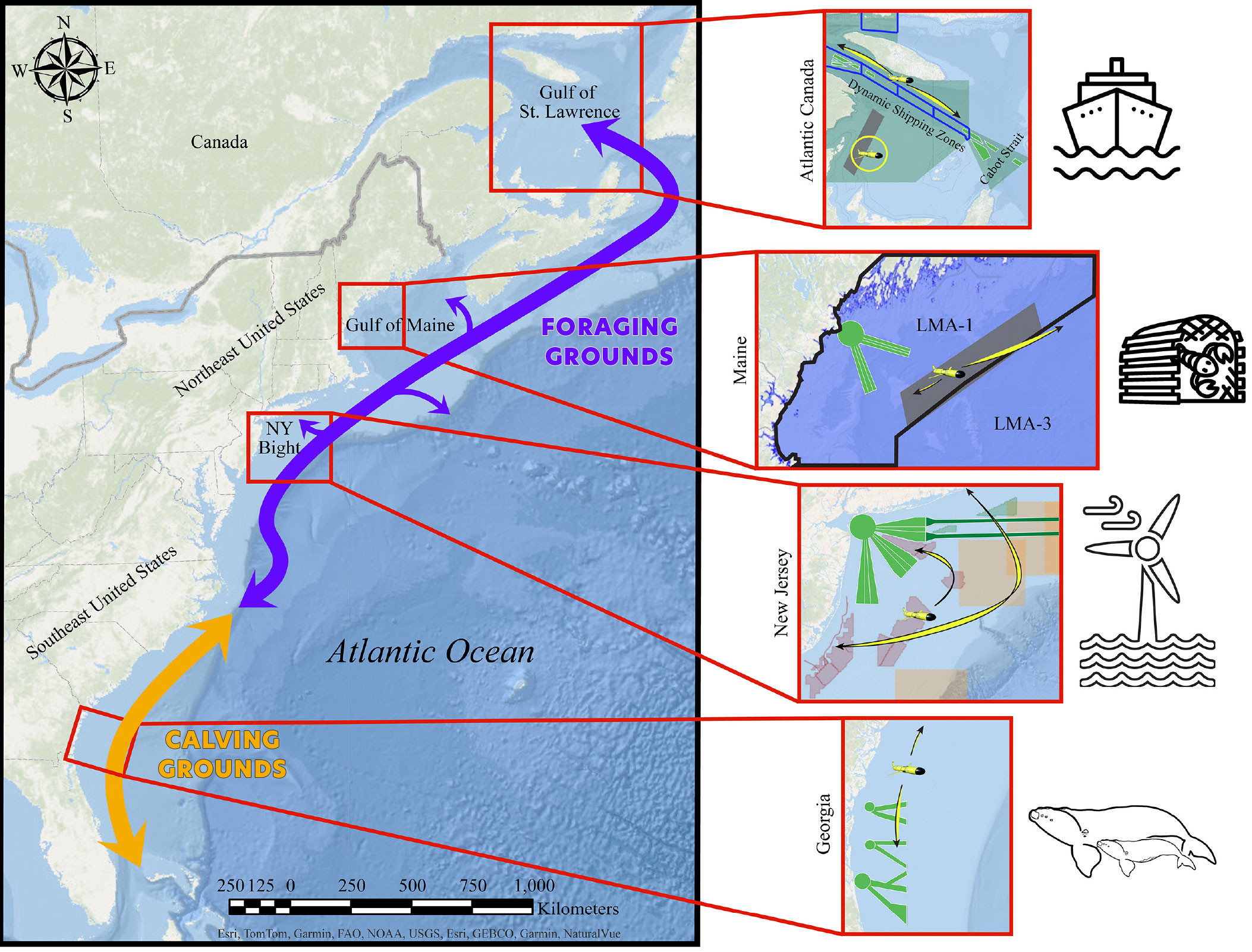
FIGURE 2. Map of the eastern United States and Canada, illustrating North Atlantic right whale (NARW) calving grounds off the southeastern United States and a foraging grounds/migratory corridor that extends along the northeastern United States into Canadian waters. Insets highlight the different regions where gliders are being used for area-based monitoring of NARWs. In Atlantic Canada, glider-derived NARW detections are used in the dynamic management of shipping zones (outlined in blue) and contribute to fishery area closures in the southern Gulf of St. Lawrence (GoSL, yellow circle); the green shading indicates all of Transport Canada’s vessel traffic management areas, which include a restricted area in the southern GoSL (gray shading), a voluntary seasonal slowdown zone in the Cabot Strait to the southeast of the dynamic shipping zones, and static 10-knot speed zones to the north and south of the dynamic shipping zones. In the Gulf of Maine, nine years of glider deployments provided insight on seasonal patterns of NARW presence, which informed the establishment of the region’s seasonal restricted area (gray shading) within Lobster Management Area 1 (LMA-1, outlined in black), where ongoing missions continue to monitor NARW occurrence. Glider missions in the New York Bight play an important role in assessing NARW habitat use relative to offshore wind energy development in the northeastern United States, with deployments in wind planning areas (shaded green polygons), wind lease areas (shaded red polygons), and busy shipping lanes (green outlines), as acoustic detections supplement visual observations in triggering dynamic voluntary slow zones (shaded orange squares). Lastly, in US southeast waters, glider deployments off the coast of Georgia contribute to an early warning system in and around heavily trafficked shipping lanes (green outlines) to mitigate the threat of vessel strike for female NARWs and their newborn calves. Yellow arrows in each inset panel indicate the general geographic span of glider missions conducted in that region. > High res figure
|
Vessel slowdowns are implemented or can be extended by regulators when a NARW is detected acoustically (via glider) or visually (via aerial surveillance) within or near a dynamic shipping zone. Slowdowns are initially triggered for a period of 15 days and apply to all vessels >13 m transiting within the active slow zone. If a speed limit is already implemented when a new detection is made, the speed limit is reset for an additional 15-day period starting on the day of the new detection, given that it occurs in the last seven days after the start of the previous slowdown (Transport Canada, 2024). When no speed restrictions are in place in the dynamic shipping zones, vessels can transit at a safe operating speed, which may vary depending on the type of vessel. Most commercial vessels normally transit at speeds over 10 knots.
Over the first four years of this dynamic management plan, there were 30 days with near-real-time acoustic detections of NARWs made during 580 glider survey days in the GoSL, triggering 194 days of dynamic shipping zone slowdowns. We found a high degree of interannual and seasonal variation in NARW acoustic occurrence that likely reflected their transitory use of the shipping lanes, as well as within- and between-season shifts in distribution across the region (recent work of author Indeck and colleagues). Gliders triggered more slowdowns than aerial surveillance by a factor of two to five during fall and summer but were less effective during spring, as whales migrating into the GoSL tend to call at lower rates and occur at lower densities than during other behavioral states (Parks et al., 2011; Matthews and Parks, 2021).
Canadian Dynamic Fishing Areas
In 2018, Fisheries and Oceans Canada (DFO) initiated a new fishery management plan to mitigate entanglement harm to NARWs from fixed-gear fisheries (primarily snow crab, Chionoecetes opilio, and lobster, Homarus americanus) in Canadian NARW habitats. Measures included mandatory static zones starting in 2018 and 2019, and dynamic fishery-area closures that began in 2020, supplemented by increased visual and acoustic survey efforts to detect NARW presence, including the use of Slocum acoustic gliders (Fisheries and Oceans Canada, 2023).
Under the current plan, the GoSL is subdivided into 10 minutes latitude × 10 minutes longitude grids. If any NARW is detected within a grid by any monitoring platform (vessel, airplane, buoy, glider, or validated opportunistic sighting), a temporary closure area is triggered for a period of 15 consecutive days, including a minimum 48-hour gear removal period (Fisheries and Oceans Canada, 2024). Each closure area is a 3 × 3 grid unit that includes the surveyed cell (i.e., the grid containing the NARW detection) and eight surrounding buffer grids, totaling approximately 2,000 km2. Buffer grids are included in the trigger to account for NARW movement after the detection is made, because NARWs can travel 80 km d–1 on average (Baumgartner and Mate, 2005). DFO is then responsible for surveying the closure area with an aerial platform during the 15-day closure. If an NARW is not detected again, visually or acoustically, within the closure area during days 9–15 and after two clearance flights (on separate days) with two trained Marine Mammal Observers on board have been completed, then the area is reopened to fishing on day 16. However, if an NARW is detected within the closure area during days 9–15, the area is put under a seasonal closure, effectively ending fishing in that area for the rest of the monitoring season on November 15 (Fisheries and Oceans Canada, 2024).
Gliders have been used to trigger fishery-area closures in the GoSL each year since 2020. During the first four years (2020–2023), the gliders triggered 13, 46, 21, and 48 grid closures, respectively, comprising a total closed area of approximately 8,700 nm2 (30,000 km2) across years. Both the DFO fisheries and the Transport Canada shipping management plans have been reviewed and adapted every year, as more has been learned about NARW presence and distribution in the GoSL.
US Monitoring to Mitigate Fishing Gear Entanglements and Vessel Strikes
Glider-based monitoring of NARWs in US waters serves several purposes, including informing mitigation efforts for fishing gear entanglements and vessel strikes. Near-real-time acoustic detections of NARWs from gliders began in the Gulf of Maine in 2012 (Baumgartner et al., 2013), in a region that in 2021 was designated a seasonal restricted area within Lobster Management Area 1, where traditional fixed-gear fishing with vertical lines is now prohibited annually from October 1 to January 31 because of the seasonal presence of NARWs (Figure 2). Glider-based detections from regular surveys of NARWs conducted by the Woods Hole Oceanographic Institution and The University of Maine were used, in part, to justify this restricted area, as well as to defend its existence in US federal court (Bowling, 2022).
Vessel strike mitigation in the United States currently consists of mandatory vessel speed restrictions in relatively small static management areas for vessels with lengths over 19.8 m, and voluntary vessel speed restrictions dynamically triggered by visual or acoustic detections of NARWs outside of the static management areas. Speed in both areas is limited to 10 knots, and dynamic management areas persist for 15 days. The program to encourage cooperation with voluntary vessel speed restrictions based on near-real-time acoustic detections was established in late 2020 and is called the National Oceanic and Atmospheric Administration’s Slow Zones for Right Whales. In the four years since its inception, 154 Slow Zones have been triggered or extended by acoustic detections of NARWs, and 51 (33%) of those Slow Zones were triggered or extended by gliders operated by the Woods Hole Oceanographic Institution, Rutgers University, Stony Brook University, and The University of Maine during 57 separate glider missions. The remaining Slow Zones were triggered by moored buoys operated by the Woods Hole Oceanographic Institution and carrying the same DMON/LFDCS system as the gliders (Baumgartner et al., 2019).
US Offshore Wind Development Areas
In pursuit of ambitious renewable energy targets, the United States plans to develop its eastern seaboard with offshore wind energy farms over the upcoming decade. Lease areas in northeastern US waters are in various stages of turbine installation, and there is a coordinated effort between the Bureau of Ocean Energy Management (BOEM), state agencies, wind energy developers, and the scientific community to address the ecological impacts of offshore wind energy development (OWD; Van Parijs et al., 2021). These impacts are anticipated to span the marine food chain through nuanced linkages between the hydrodynamics and food web ecology at turbine, wind energy area, and regional scales (NASEM, 2024).
Within the US Northeast, increases in NARW occurrence have been observed south of traditional foraging grounds in the Gulf of Maine since approximately 2010, in regions where considerable OWD is ongoing or upcoming (Davis et al., 2017; Meyer-Gutbrod et al., 2022). Further, OWD is occurring in regions such as the New York Bight, which has historically received limited survey effort and has lacked density estimates and detailed distributional data for large whales until recently (Zoidis et al., 2021). PAM-equipped gliders operated by Rutgers and Stony Brook Universities are playing a key role in assessing the habitat use of NARWs and other large whales relative to OWD in the northeastern United States.
Since 2020, gliders have surveyed for over 700 days and have transited more than 14,000 km in and adjacent to wind lease areas in New York and New Jersey (Figures 2 and 3). These surveys have documented detections of NARWs on 10%–20% of survey days from November to March, and <5% of survey days from March to October. Continued monitoring, and the comparison of pre- and post-development occurrence data in OWD areas, will allow potential impacts of OWD on NARWs to be assessed. Further, by providing NARW detections, along with concurrently sampled subsurface oceanographic data, glider surveys will help to improve our understanding of the environmental drivers of NARW habitat use in these previously understudied regions. Given the rapid environmental change occurring in the northeastern United States, this information will be critical to distinguishing impacts of OWD on habitat use from effects of environmental variability.
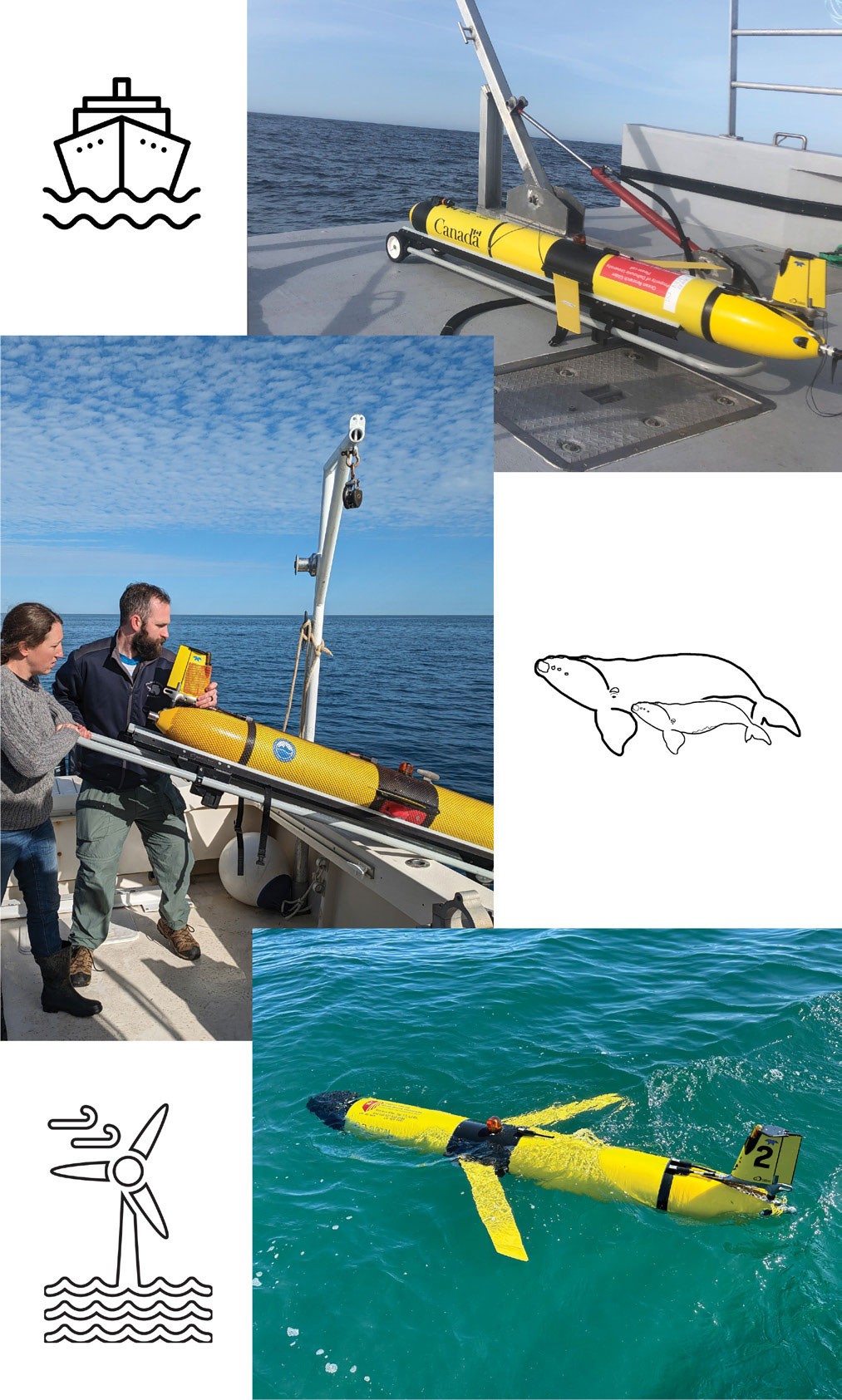
FIGURE 3. Photos of gliders in the field show them, from the top, ready for deployment in the Gulf of St. Lawrence, Canada, being deployed by field personnel on the coastal calving grounds of Georgia, USA, and in the water during deployment in the New York Bight, USA. > High res figure
|
US Coastal Calving Ground Surveillance
Pregnant NARWs migrate to nearshore southeast US calving grounds, spanning the states of Florida, Georgia, and South Carolina, to give birth and nurse their newborn calves between the months of November and April (Gowan and Ortega-Ortiz, 2014). Non-reproductive individuals also migrate to this calving ground during the winter months (Gowan et al., 2019). Although gliders have been used for near-real-time detections of NARWs in northern foraging grounds off the coast of the United States and Canada for more than a decade, gliders were not used until 2023 for PAM in the southeast US calving ground, where glider-based PAM faces two major challenges. First, vocalization rates of lactating females compared to other demographic groups are lower in the calving ground (Parks et al., 2019b), and these calls tend to be low amplitude (Parks et al., 2019a). This reduces the likelihood of acoustically detecting a mother-calf pair in this region. Second, the calving ground is situated close to the coast over a shallow portion of the inner continental shelf. Typical depth occupancy in the calving ground is between 10 m and 25 m, which provides limited vertical space for a glider to operate its dive-climb flight pattern. The frequent shift between ascending and descending status requires more frequent engagement of the buoyancy pump, which is both energetically costly and produces self-noise that may mask whale vocalizations. Further, strong gradients in temperature, salinity, and thus sound speed, may further limit detection range.
Despite these challenges, a pilot program using gliders for NARW PAM was recently implemented through a collaboration between the University of South Carolina and the Skidaway Institute of Oceanography. So far, these efforts consisted of a two-week mission in January 2023, and two four-week missions from January through March 2024 (Figures 2 and 3). During these three missions, which operated in water as shallow as 11 m, three definite NARW detections were made. The NARW detection on January 20, 2024, was the first definite glider-based acoustic detection in southeast US waters, and it triggered an alert from the Southeast Early Warning System notifications program for vessel strike mitigation. These pilot missions indicate that glider-based PAM may be a useful tool for supplementing aerial-based detections of NARWs in the calving ground, especially providing coverage when aerial surveillance is not feasible due to weather or other logistical constraints.
Conservation Implications
North Atlantic right whales are at risk of extinction before the end of the century, as climate change continues to initiate distributional and behavioral changes that inadvertently increase mortality due to vessel strikes and entanglements (Meyer-Gutbrod et al., 2021). As a result, the Canadian and US governments are investing millions of dollars in technologies to support species monitoring, research to better predict future whale distributions, and mitigation efforts to address complex threats to vulnerable species. In the last five years, glider efforts have rapidly expanded, with cumulative deployments totaling thousands of days across the NARW migratory range for conservation applications (Figure 3). Here, we highlighted several examples of how PAM-equipped underwater gliders are being used for vessel strike and entanglement mitigation by enhancing risk reduction for dynamic management areas, regional fisheries, and offshore wind energy projects (Table 1). Going forward, this technology has the capacity to contribute to more spatial conservation strategies, such as marine protected areas. These areas tend to encompass vast and/or remote areas that are logistically difficult for personnel to survey, which can hamper authorities’ ability to enforce protective measures. Therefore, the use of gliders is likely to continue expanding into the future and across the marine domain in support of species conservation and threat mitigation.
Glider-based PAM offers key spatial monitoring capabilities for NARW threat management but is typically used in conjunction with other, complementary monitoring platforms, such as aerial surveillance and PAM moorings. Because NARWs are an internationally mobile cetacean species that spans diverse habitats and protection measures, surveillance assets must fulfill different requirements (e.g., temporal effort, spatial scale, deployment location, data type) across the NARW range, depending on the goal(s) of each individual monitoring program. Because the space-time needs of successful range-wide management are so complex, no one tool could possibly achieve all monitoring imperatives. As one example, gliders were deployed in the Cabot Strait voluntary seasonal slowdown zone in the GoSL for two years. Despite being a known high-use migratory corridor, we did not acoustically detect any NARWs in near-real time. This may have been because of behaviorally influenced calling rates, missed whales traveling close to shore (i.e., deployment location vs. whale movements), or platform type (e.g., mid-endurance mobile glider vs. long-endurance stationary array). Thus, glider-based PAM is being used alongside a suite of other tools, including moored PAM, visual monitoring, and distribution modeling, to aid conservation goals.
We have presented several different area-based threats, management goals, and mitigation plans across glider survey regions. These highlight the need for continued research/support for additional and/or new monitoring platforms (including gliders) to be incorporated into existing and future management plans for the conservation of NARWs. However, the efficacy of glider detections at achieving conservation goals depends largely on the regional regulatory measures in place being informed by these detections. For example, in a recent study, the average percentage of mariners found to be cooperating with 10-knot speed requests in US dynamic management areas was less than 50%, compared to higher compliance in some, but not all, mandatory seasonal management areas (>85% in most areas, but <25% for the largest commercial vessels outside four ports in the southeastern United States; NMFS, 2020). In contrast, Canada has made mitigation measures in the dynamic shipping zones of the GoSL mandatory and achieved 99% compliance during the 2023 monitoring season (Bilodeau, 2023). Furthermore, slowdowns in eastern Canadian waters affect vessels down to 13 m, whereas US speed restrictions currently only apply to vessels that are greater than or equal to 19.8 m. This difference is significant, as four of 13 vessel-related NARW deaths in US waters since 2008 were attributable to boats less than 20 m and, therefore, not subject to slowdown measures (Redfern, 2023). No matter how capable a technology or monitoring system, its overall conservation impact is intertwined with the prevailing management policies.
Acknowledgments
We would like to thank the following for funding support: Transport Canada, Fisheries and Oceans Canada, the Ocean Tracking Network, Marine Environmental Observation, Prediction and Response Network, the Murphy Family Foundation, the Broad Reach Fund, NOAA Northeast Fisheries Science Center, the Northeastern Regional Association of Coastal Ocean Observing Systems, the New York State Environmental Protection Fund Ocean and Great Lakes Program via the New York State Department of Environmental Conservation, the New York State Energy Research and Development Authority, the Mid-Atlantic Regional Association Coastal Ocean Observing System, the Tides Foundation, and the Southeast Coastal Ocean Observing Regional Association.
We would also like to express our sincere appreciation to the following individuals, who were key to glider operations and mission support: A. Comeau, J. van der Meer, Z. Viva, B. Hodges, M. Meier, J. Wilder, C. Flagg, D. Aragon, N. Waite, J. Kohut, T. Miles, A. Kreuser, A. Sefah-Twerefour, K. Dreger, J. Bird, F. McQuarrie, and A. Vincent.