Introduction
Organisms and populations depend on each other. When one species is harmed or perturbed, other species can be affected, and connections that bind species together to make functional ecosystems can be stressed or broken. The Deepwater Horizon (DWH) oil spill was unprecedented in the vastness of the area impacted (149,000 km2), duration (87 days), and amounts of pollutants added to the environment (approximately 635,000,000 liters of oil and 25,740,000 liters of dispersant; see Rullkötter and Farrington 2021, in this issue). Uncountable species were affected, profoundly altering the complex interactions that ecosystems rely upon to remain resilient. Whereas some impacts of the DWH spill on the environment and ecosystem services were easily discernible and quickly recovered, a far greater number of effects have been hard to measure, assess, and even observe. Nonetheless, one of the major endeavors of the Gulf of Mexico Research Initiative (GoMRI) effort was to elucidate how the DWH spill impacted the patterns and trends of perturbations, responses, and recoveries in the multiple ecosystems that comprise the Gulf of Mexico region. Here, we highlight some of the significant findings and questions that emphasize needs for future consideration at population, ecosystem, and community levels.
The Gulf of Mexico hosts a diverse set of ecosystems that intersect at numerous levels of scale and complexity (Paris et al., 2020). In some cases, the boundaries between habitats, communities, and ecosystems can be sharp, as is the case between high beach and intertidal regions. In other environments, transitional boundaries can be blurred, for example, between nearshore and deep benthic habitats. Here, we consolidate habitats impacted by the DWH spill into four major environments: onshore, coastal, open ocean (shallow to deep water column), and deep benthos.
Considerable research attention has been given to these systems, but the amounts of effort and types of research have varied greatly, in part because our baseline knowledge prior to the oil spill varied among habitats. In the case of open ocean or deep benthic habitats, we are still discovering which taxa are present and we have limited knowledge of species-species interactions (Schwing et al., 2020; Sutton et al., 2020). In contrast, the biodiversity of coastal and marsh areas is reasonably well known, with some understanding of ecosystem functions (Mendelssohn et al., 2017; Murawski et al., 2018, 2021, in this issue). What we have learned about various habitats is summarized elsewhere (Mendelssohn et al., 2012; Rabalais and Turner, 2016; Powers et al., 2017; Rabalais et al., 2018; Schwing et al., 2020). Here, we examine findings that transcend habitat or ecosystem boundaries and also explore significant insights gained for particular environments.
Variable Timescales of Impacts
Consistent with previous research on oil spills (e.g., Peterson et al., 2003; Lincoln et al., 2020), available evidence suggests that impacts of the DWH spill on Gulf of Mexico biodiversity and ecosystem functions will last for many decades. In places like salt marshes and deep benthic environments, hydrocarbons persist, as removing them was impossible. In general, toxicants left systems in one of three ways: (1) hydrocarbons and dispersants, and their subsequent by-products, were metabolized, although some of them were incorporated into organismal cells; (2) weathered residues containing hydrocarbons and other products were, and are being, buried to the point that associated chemical moieties are either no longer available to organisms (assuming no disturbance) or have spatially localized impacts; and (3) toxicants were actively removed from the system either by processes such as advection (e.g., water movement, organismal transport) or human intervention (e.g., beach cleaning). Of course, some environments facilitate relatively rapid metabolic consumption or human removal (e.g., surface waters and nearshore environments), whereas deposition, burial, and negligible degradation may dominate in inaccessible deep benthic and some marsh environments. In different habitats or ecosystems, these mechanisms work on spatial and temporal scales that vary considerably, making it nearly impossible to accurately answer the seemingly simple question: how long will the DWH impacts last?
One of the first notable changes in ecosystems was the uptake of Macondo hydrocarbons into the pelagic microbial food web and subsequent uptake by eukaryotic organisms via feeding (Figure 1). This pulse of carbon energy happened in both the nearshore (Graham et al., 2010) and the open ocean (Chanton et al., 2012). Although this pulse was ephemeral in nature and waned as the carbon source was altered or exhausted, it supplied energy at the base of the food chain that was distinctly different than typical photosynthetic carbon fixation pathways to which most ecosystems are finely tuned. The initial increase in microbial carbon fixation was relatively short lived, but the movement of the carbon pulse through various trophic levels can take years, with impacts dissipating as it moves to higher levels (Patterson et al., 2020). Importantly, the spill did not just introduce pollutants, it fundamentally perturbed the base of the food web. Thus, as long as DWH hydrocarbons are present, environments have the potential to be impacted from both toxicant effects and an altered carbon cycle (Peterson et al., 2003; Lincoln et al., 2020). For example, in 2012, Hurricane Isaac resuspended previously buried DWH hydrocarbons sequestered in nearshore sediments (Diercks et al., 2018).
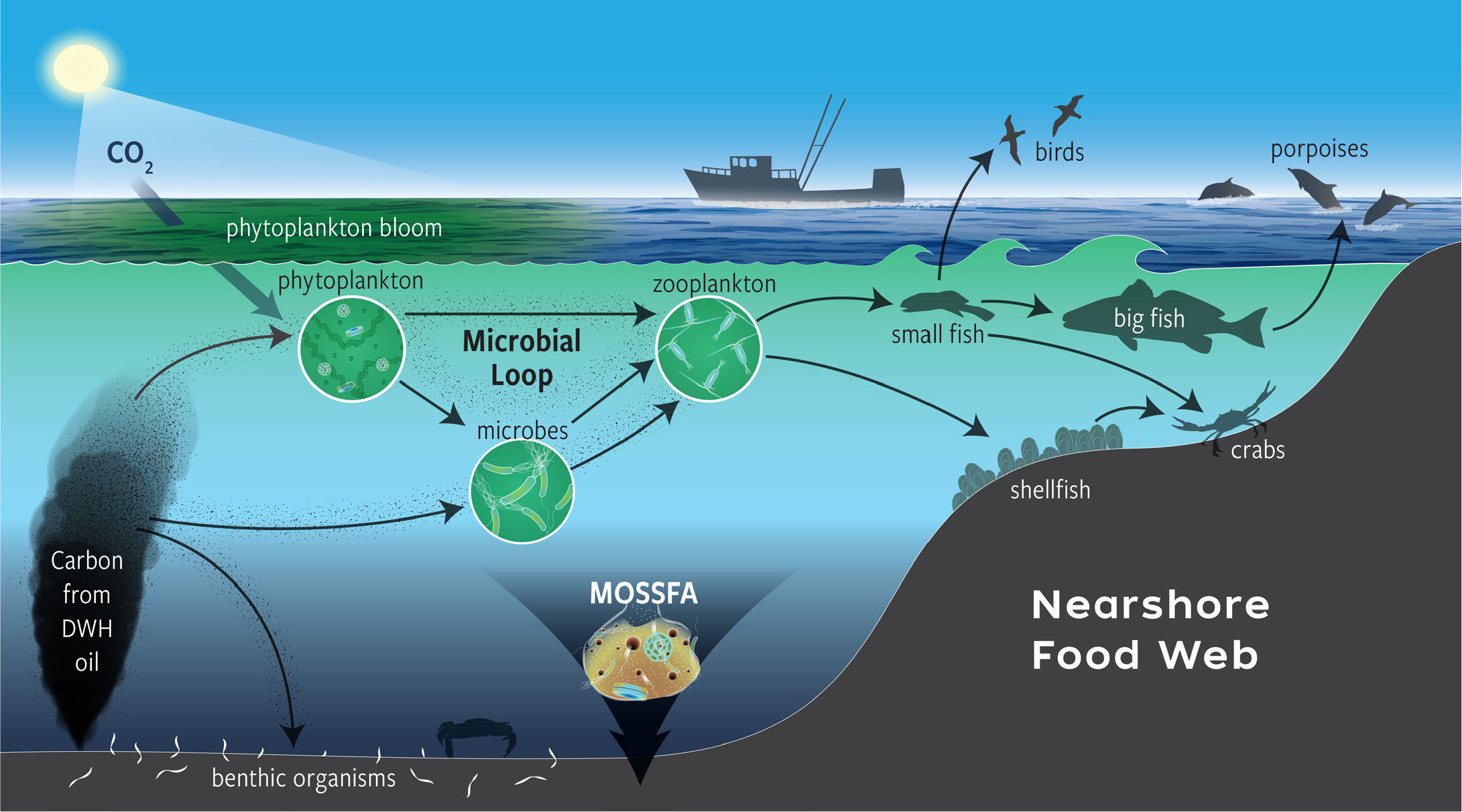
Figure 1. Hydrocarbons from the Macondo wellhead were incorporated into the pelagic microbial food web and subsequently passed on to eukaryotic organisms via food web interactions. Hydrocarbons moved into both coastal and deep benthos ecosystems. > High res figure
|
Variation in responses to the DWH spill by different environments was caused not only by the different amounts of oil received in any particular location but also by the duration pollutants were retained in the system and whether the system was of high or low productivity. Beaches, wetlands, and deep benthos serve as three illustrative examples of how the influence of hydrocarbon burial or removal can proceed on very different timescales (Figure 2).
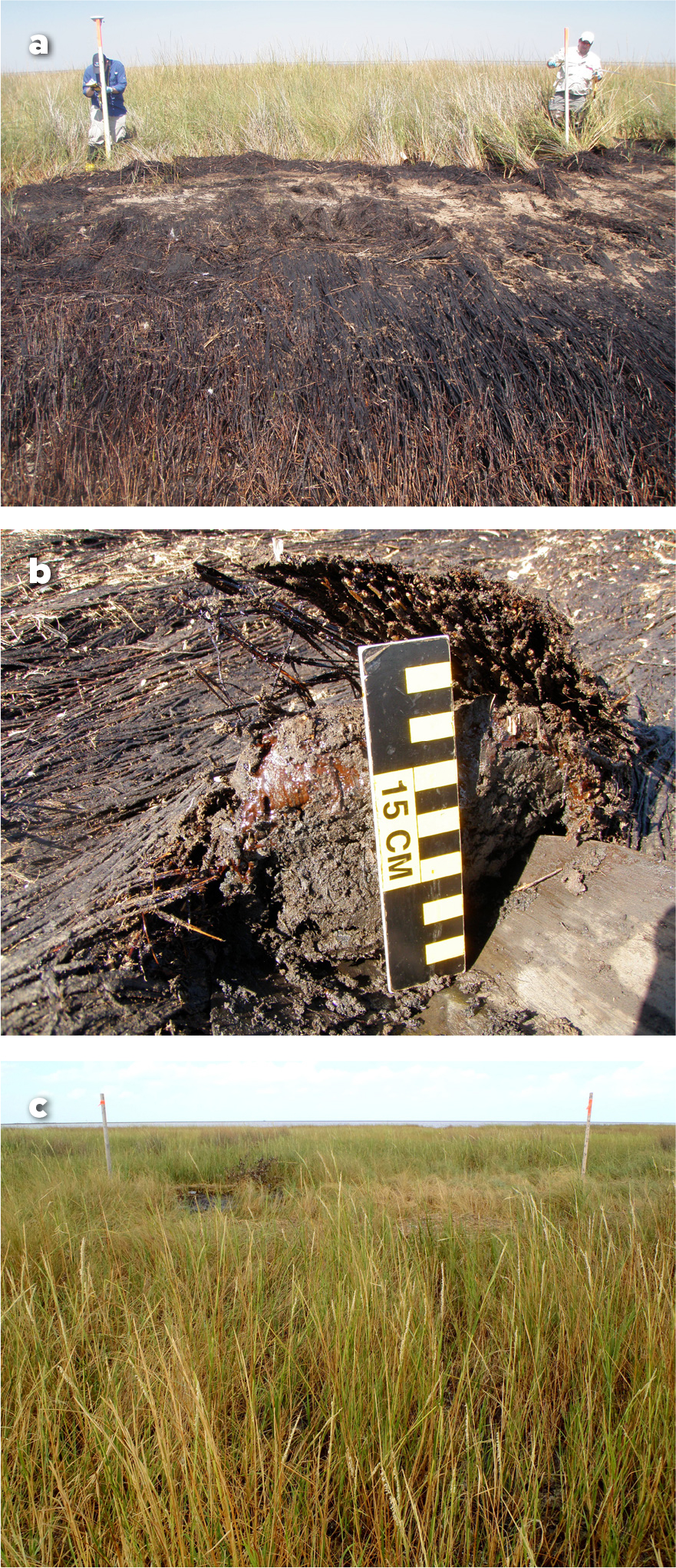
Figure 2. Photos of heavily oiled wetlands along Barataria Bay. See title page photo for aerial view (a) A ground-level plot exhibits the extent and ingression of bulk oiling. (b) This close-up photo reveals oil thickness on the substrate (~2–3 cm). (c) Recovering vegetation is shown about two years after manual cleanup. Photos from Zengel et al. (2015). > High res figure
|
DWH oil deposited on Florida sandy beaches in June 2010 was rapidly buried, contaminating the sediment down to 70 cm depth (Figure 3). Degradation rates scale with the kinetic and radiant energy present, so oil deposited on Gulf sandy beaches decomposed more rapidly than oil in the deep sea. Hydrocarbons in small (millimeter-size) sand-oil agglomerates were degraded within a year by a diverse microbial community, boosted by warm temperatures and aerobic conditions maintained by tidal pumping of oxygen through the contaminated sediment (Huettel et al., 2018). In some cases (e.g., Pensacola, Florida), excess hydrocarbons caused blooms in bacterial biomass (Kostka et al., 2011) that favored hydrocarbon-degrading microbes, leading to a drastic temporary decline in taxonomic diversity (Rodriguez-R et al., 2015; Gao et al., 2018). Chemical evolution of hydrocarbons can drive succession of microbial species, and once most of the oil was degraded, microbial communities returned to near baseline abundances, with little evidence of being enriched for hydrocarbon-degrading bacteria (Kostka et al., 2020).
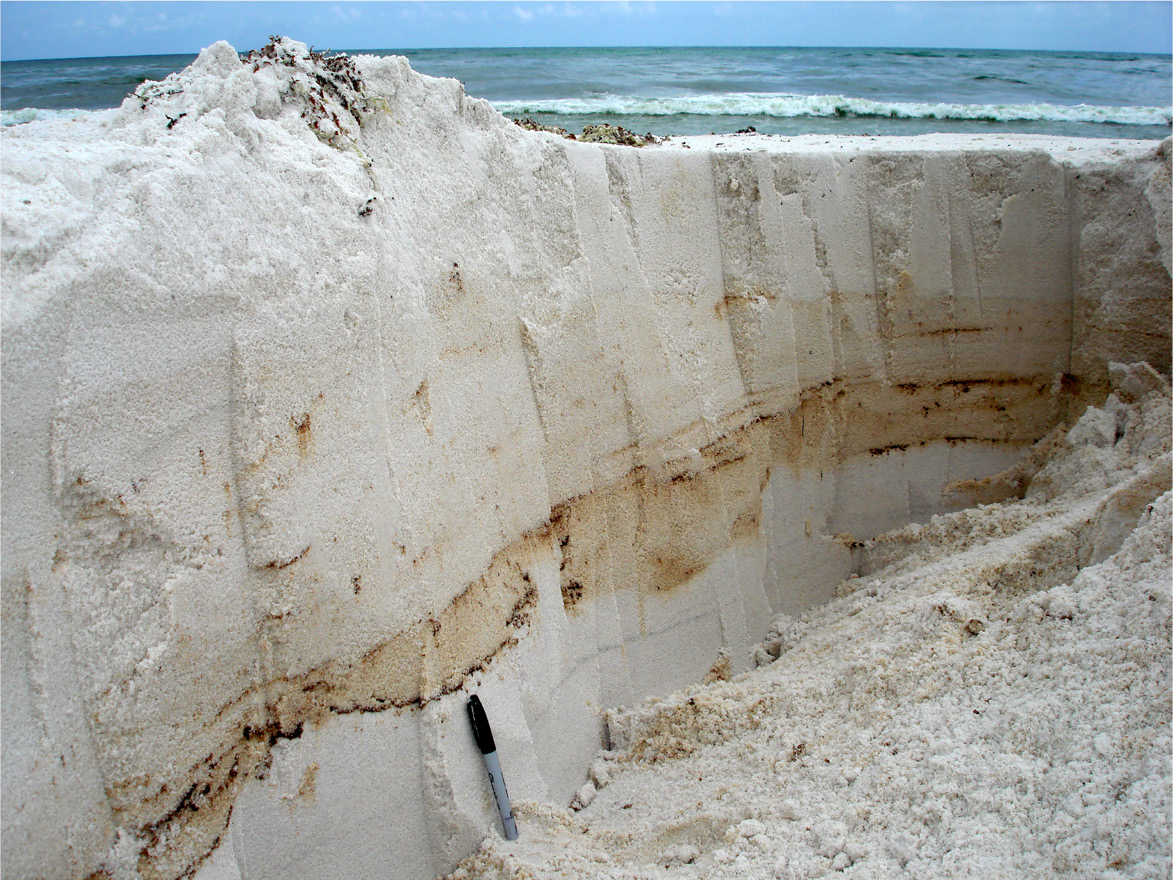
Figure 3. Cross section of sand at Pensacola Beach, Florida, photographed on July 25, 2010. The dark double layer produced by oil deposited during the nights of June 22 and June 23 is visible at 45–50 cm depth. Oil was buried in Pensacola Beach sands down to ~70 cm depth. Note the marker for scale. Photo credit: Markus Huettel. > High res figure
|
In wetlands, oil impacts were generally limited to salt marshes bordering estuarine bays and barrier islands as well as low-salinity reed swamps of the Mississippi River birdfoot delta (Mendelssohn et al., 2012; Michel et al., 2013). Effects on wetland vegetation were highly variable and dependent upon oiling intensity and penetration of oil inland, among other factors. Along heavily oiled shorelines, oil frequently pooled under vegetation and often penetrated the soil more deeply through crab burrows (Zengel and Michel, 2013). Highest concentrations of oil were found in the top 2 cm of the soil profile but penetrated to 8 cm depth (Atlas et al., 2015) and possibly deeper (Natter et al., 2012). Heavily oiled marshes suffered combined effects of toxicity and smothering. In general, plant survival, growth, and recovery depended on oiling severity, duration, and species affected, with impacts decreasing with distance from oiled shorelines (Silliman et al., 2012; Zengel et al., 2015; Chen et al., 2016; Hester et al., 2016; Lin et al., 2016; Beland et al., 2017). Differential species tolerance to Macondo oil was particularly evident. For example, black needlerush (Juncus roemerianus) was more sensitive and less resilient to oiling than smooth cordgrass (Spartina alterniflora; Lin and Mendelssohn, 2012; Lin et al., 2016). Along some shorelines, recovery was likely impaired or prevented by shoreline erosion, which oiling can accelerate (Zengel et al., 2015; Hester et al., 2016; Beland et al., 2017). On a positive note, vegetation that was lightly or moderately oiled by the DWH spill showed little impact or recovered rapidly (Lin and Mendelssohn, 2012; Hester et al., 2016; Lin et al., 2016). In fact, a case study of heavy oil exposure from a pipeline rupture of Louisiana crude oil (pre-DHW) documented near complete recovery of Spartina after four years (Hester and Mendelssohn, 2000). Lin et al. (2016) estimated full vegetation recovery of heavily oiled marshes from the DWH spill may take five years or longer, assuming minimal shoreline retreat.
Deep-sea hydrocarbon degradation models suggest that recalcitrant residues will remain in sediments for about a decade (Romero et al., 2017). Pre-DWH spill abyssal sediment accumulation rates ranged from 0.04–0.44 cm yr–1 and bioturbation depths ranged from 1.75–3.25 cm (Yeager et al., 2004). Mass accumulation rates of sediment increased four- to tenfold during and immediately following the DWH spill (Brooks et al., 2015), with a concomitant decrease in abundances of meiofaunal and macrofaunal densities by as much as 90% in some areas (Schwing et al., 2015; Montagna et al., 2017). Such massive sedimentation rates, toxicant input, and fauna decreases have major impacts on ecosystem function and, importantly, will directly impact benthic-pelagic coupling of nutrient cycles and food chains. Taking all of these factors into account, 50–100 years will be needed to achieve full recovery from DWH contaminant burial below bioturbation depths (Schwing et al., 2020).
In all three of these environments, oil is being sequestered by burial. Whereas oil per se in the open water column was not retained for a long time, the bioavailable hydrocarbons and hydrocarbon pollutants have had a long-lasting impact. There is evidence that as late as 2017, DWH-derived toxicants were measurable in eggs of pelagic fishes and shrimps at levels above those known to cause sublethal effects (Romero et al., 2018). Though life histories, including longevity, of deep pelagic organisms are poorly known, extrapolation from known fauna suggests that individuals examined were several generations removed from the spill, indicating DWH-derived residues have persisted in the deep pelagic environment. These long-term elevated concentrations may be a result of food-web incorporation (Graham et al., 2010; Chanton et al., 2012; Quintana-Rizzo et al., 2015) and/or the persistence of oil residues in the water column (Walker et al., 2017) due to resuspension of contaminated sediments (Romero et al., 2017; Diercks et al., 2018), providing one possible explanation for widescale persistent declines in mesopelagic population abundances in the Gulf after the DWH spill (unpublished data of author Sutton).
Prolonged Impacts Versus Fast Recovery
Impacts of environmental insults can be spatiotemporally delayed in part because biological processes (e.g., behavior, physiology, ontology, recruitment) can operate on long timescales (Peterson et al., 2003). Determining the timing and magnitude of delayed impacts, as well as the ecological mechanisms that propagate the impact, can be exceedingly difficult even for relatively simple biological systems. However, observations made since DWH confirm ecological theory as to how different trophic levels may be impacted by an environmental disturbance such as an oil spill.
Upper trophic levels can exhibit considerably delayed impacts from disasters and toxicants relative to lower trophic levels, which tend to be short-lived species with higher fecundity and abundances. Depending on the situation, life-history variables can act in concert, or independently, to moderate or exacerbate impacts from an oil spill. Factors such as body size may influence how much toxicant needs to be absorbed to produce detrimental effects, or motility may influence how often or for how long an organism comes into contact with a pollutant. Such factors will influence the rate and magnitude of toxicant damage that can lead to death, short-term sublethal impacts, or long-term persistent damage. The 1989 Exxon Valdez oil spill in Prince William Sound offers an instructive example of chronic exposure. It exacted a sublethal toll on the sea otter (Enhydra lutris) population, which failed to recover and indeed declined over a prolonged period within the oiled area (Peterson et al., 2003). In the case of the DWH spill, higher trophic levels tend to show more prolonged impacts and slower rates of recovery (this is in part due to bioaccumulation impacts of polycyclic aromatic hydrocarbons, or PAHs, in fatty tissue; see Murawski et al., 2021, in this issue). Additionally, because organisms at higher trophic levels have generally smaller population sizes and longer generation times, obtaining appropriate numbers to allow for statistically rigorous analyses on the impacts of sublethal effects can be difficult. The bottlenose dolphin (Tursiops truncates) exemplifies impacts on a top predator in the Gulf of Mexico system. Dolphin populations are highly vulnerable to hydrocarbon impacts and have medium to low resilience, limiting their ability to recover (Murawski et al., 2020).
In contrast, lower trophic levels tend to show more resilience because they typically have short generation times and high fecundity, allowing faster replacement of individuals, especially among species, or genotypes within species, that may be competitively superior given the altered environmental conditions. For example, many nearshore commercial shellfish communities rebounded fairly quickly in the wake of the DWH spill (Gracia et al., 2020). Presumably, their resilience is due in part to their reproductive biology. For example, penaeid shrimp (Farfantepenaeus aztecus, F. dourarum, and Litopenaeus setiferus) and blue crab (Callinectes sapidus; Figure 4) lay a larger number of eggs and mature within one to two years.
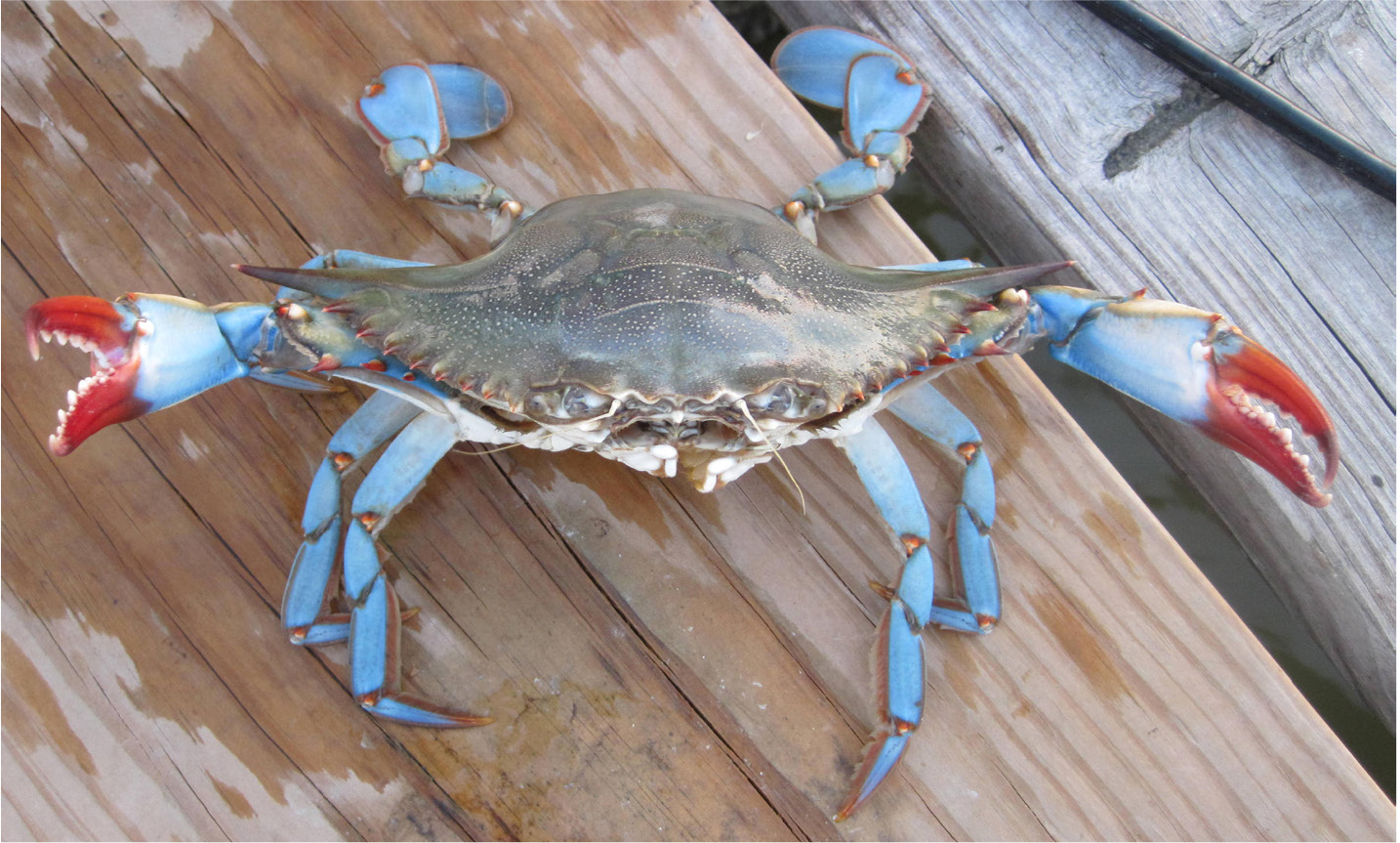
Figure 4. The blue crab, Callinectes sapidus (whose names means “tasty beautiful swimmer”), is an example of a species that seemed to recover quickly after the spill, in part because of its high fecundity. Photo credit: Bree Yednock. > High res figure
|
Although the speed with which a population of a given species may recover is roughly correlated with trophic level and fecundity, how quickly the overall ecosystem recovers is more closely aligned with available energy, which influences productivity. Organisms living in high productivity environments, such as salt marshes or the epipelagic water column, which both receive large inputs of solar energy, recovered faster because more energy was available for growth, repair, and reproduction. In comparison, energy is scarce in deep environments. Metabolic rates in deep benthic organisms are slower than those in the pelagic zone due to limited food resources and cooler temperatures, and thus turnover rates for larger deep benthic organisms (macrofauna, megafauna, corals) are generally slower than those of smaller, shallow benthic organisms (Rowe and Kennicutt, 2008; Montagna et al., 2017). For example, estimates of deep coral growth range from 0.03 cm yr–1 to 0.2 cm yr–1 (Prouty et al., 2016), which corresponds to centuries of growth to achieve a colony on the scale of tens of centimeters.
As is the case with complex biological systems, outcomes can vary depending upon the species and the population. How the spill affected commercially harvested fish seems to have varied depending upon multiple factors, including fecundity, trophic position, and sensitivity to accumulated PAHs. For example, some commercial fish communities recovered quickly despite stressors from the DWH spill, but changes in fishing pressures may have influenced recovery (Gracia et al., 2020). Shortly after the spill, impacts on bluefin tuna (Thunnus thynnus) were of concern because the DHW spill disrupted their spawning season. Fortunately, <12% of bluefish tuna larvae were in contaminated waters because their spawning grounds are broadly distributed (Muhling et al., 2012), and a large impact on that population has not been observed to date (bluefin tuna take about eight years to sexually mature). Potential impacts on red snapper (Lutjanus campechanus) have also been a point of concern. These fish mature at two years and have a >50-year life span. Thus, their reproductive potential augments their resilience.
Ecosystem Engineers and Clonal Species
Although many attributes of ecosystem function are driven by energy availability or trophic level, ecosystem engineers can control environmental complexity through niche availability. In particular, three-dimensional structure, critical for both diversity and abundance of many species, seems somewhat limited in the Gulf of Mexico given the sandy shallow coastline and shelf that lack rocky substrate. Interestingly, in wetland, nearshore, and deep-sea regions of the Gulf of Mexico, organisms, rather than geological features, fill the role of providing three-dimensional structure. Structure relates to niche diversity and availability and thus biodiversity. In these systems, taxa responsible for engineering environmental structure tend to be clonal species, meaning that the organism has limited genetic variability and tends to be long-lived. These clonal species are being stressed in multiple ways as well. A major concern of the DWH spill recovery included whether the damage to ecosystem engineers would result in loss of three-dimensional habitat structure that would impact recovery of other species.
During the DWH spill, effort was made to keep oil away from nearshore and coastal areas in part to protect the sensitive nursery grounds of seagrass beds (e.g., Halodule wrightii and Thalassia testudinum) and saltmarsh wetlands (mainly, Spartina alterniflora). Spartina habitats, in particular, are critical as they also provide many ecosystem services, such as erosion control and surface and subsurface habitat; are an interface between the marine and terrestrial environments; and host considerable biodiversity. As a result of the intentional mitigation efforts, the offshore water column and deep benthos received large amounts of oil and dispersants. That decision doomed many deep-sea communities, including deep coral assemblages, some of which are estimated to be over 2,000 years old (Girard et al., 2019). Coral colonies themselves were covered to varying degrees in the flocculent material derived from marine oil snow produced by the DWH spill. Colonies that were lightly impacted have shown signs of recovery, while those with more extensive injury have continued to decline and have lost entire branches of their colonies (Girard et al., 2019). Reductions in the numbers and sizes of colonies at the impact sites will affect total reproductive output because their fecundity is dependent on the number of reproductive polyps in the colony. Coral growth rates are extremely slow (Prouty et al., 2016), and recruitment is sporadic (Doughty et al., 2014), suggesting that population recovery will be considerably delayed. Just as with shallow-water coral reefs, deepwater corals are critical for providing three-dimensional structure and increasing biodiversity. One of the most common and persistent associations is with the ophiuroid brittle stars, which were shown to accelerate the rate of coral colony recovery following the spill by preventing settling of or dislodging flocculate oil from the corals, although some of the brittle stars died as a result of their efforts (Girard et al., 2016; Figure 5). Octocoral colonies serve as nursery grounds for some fish species, including the chain catshark (Etnoyer and Warrenchuk, 2007) and Sebastes spp. (Baillon et al., 2012), as well as octopus species (Shea et al., 2018). Because of their significant role in the generation and maintenance of biodiversity through direct habitat provision and increased habitat heterogeneity (Lessard-Pilon et al., 2010), the loss of these coral structures and their associated fauna in the deep Gulf of Mexico will have repercussions for the biological and genetic diversity of these habitats on long timescales.
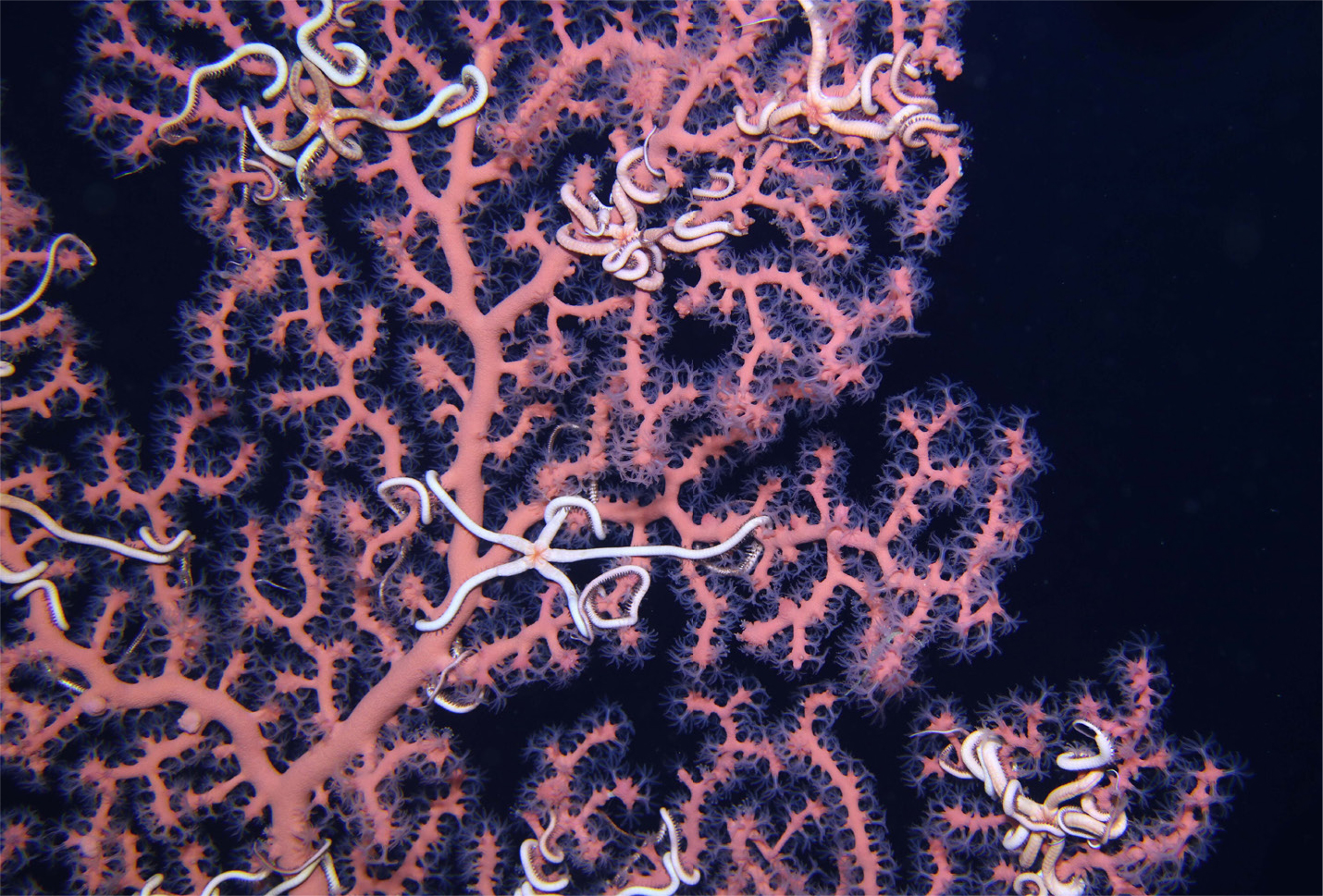
Figure 5. Brittle stars (Asteroschema clavigerum) on the deep-sea coral Paragorgia sp. In this image, both animals are healthy and demonstrate the intimate association between the two species. Brittle stars protected corals by preventing settling of or dislodging flocculate oil from the sessile organism. Photo courtesy of ECOGIG. > High res figure
|
Ecosystems are Interconnected
One surprise of GoMRI research was the degree of coupling among Gulf of Mexico ecosystems. Hydrocarbons and associated toxicants showed considerable vertical and horizontal spatial connectivity in addition to having a temporal component. As mentioned in Quigg et al. (2021, in this issue), dispersants were hard to track and, unfortunately, we have few long-term data on these chemicals. Both physical and biological processes accounted for transport of spill-related toxicants. Conventional wisdom suggests that spilled hydrocarbons, weathered hydrocarbons, and dispersants were moved by physical transport mechanisms (currents, sedimentation) and that biologically modified or incorporated products were moved between ecosystems by organisms. However, this is an oversimplification of how DWH toxicants spread throughout the Gulf of Mexico. Both physical and biological processes facilitated dispersal of hydrocarbons, dispersants, and their downstream byproducts, suggesting considerably more interdisciplinary research is needed to fully understand the magnitude and mechanisms of connectivity between ecosystems. (For discussion of the physical mechanisms that initially distributed DWH products, see Boufadel et al., 2021, and Farrington et al., 2021, both in this issue).
Once hydrocarbons and toxicants had worked their way into biological systems, migration and predatory-prey relationships promoted transfer of those chemicals to other ecosystems. For example, diel vertical migrations account for movements of organisms en masse up and down the open ocean water column in search of food. Approximately half of all fish species and three-quarters of all pelagic macrocrustaceans (shrimps, krill, and mysidaceans) in the open Gulf of Mexico vertically migrate in some form (Sutton et al., 2020), including most of the biomass-dominant taxa. This upward movement from meso- and bathypelagic depths during daytime into shallow layers at night, and the concomitant descent at sunrise, constitutes the largest synchronous migrations of animals on Earth, and, through the linked process of feeding, is a key driver of the “biological pump,” the primary mechanism whereby active animal movement sequesters carbon in the deep ocean. Given the presence of subsurface plumes of hydrocarbons and dispersants after the spill, vertical migrations by the deep-pelagic fauna increased their exposure to DWH spill contamination (Sutton et al., 2020). This active vertical flux also likely served as a vector for contaminant redistribution via plankton consumption near the surface and defecation at depth (Hopkins et al., 1996). Given that very little particulate organic carbon reaches the seafloor in the open Gulf (Rowe et al., 2008), tainted fecal pellets were likely consumed within the deep water column rather than being directly deposited and buried at the seafloor.
In addition to vertical migration, animals move horizontally to new regions to feed, shelter, or reproduce. As organisms carrying toxicants enter new areas, they may reproduce, defecate, be eaten, or die, thus releasing the byproducts of the DWH spill into novel areas (Figure 6). Knowledge of food web connection or migratory movements have been used in models to improve our understanding and ability to model the spread of toxicants (Larsen et al., 2016). Moreover, coupling between the benthos and the water column can serve to move chemicals among different reservoirs. Although processes such as sediment resuspension, sedimentation (including marine snow from biological processes), predation, and bioturbation are well understood, GoMRI research has highlighted the role these processes play in facilitating the movement of hydrocarbons. The most significant of these coupling events was the large amounts of hydrocarbons and tainted marine snow sediments that were deposited on the deep benthos, mentioned above (Vonk et al., 2015).
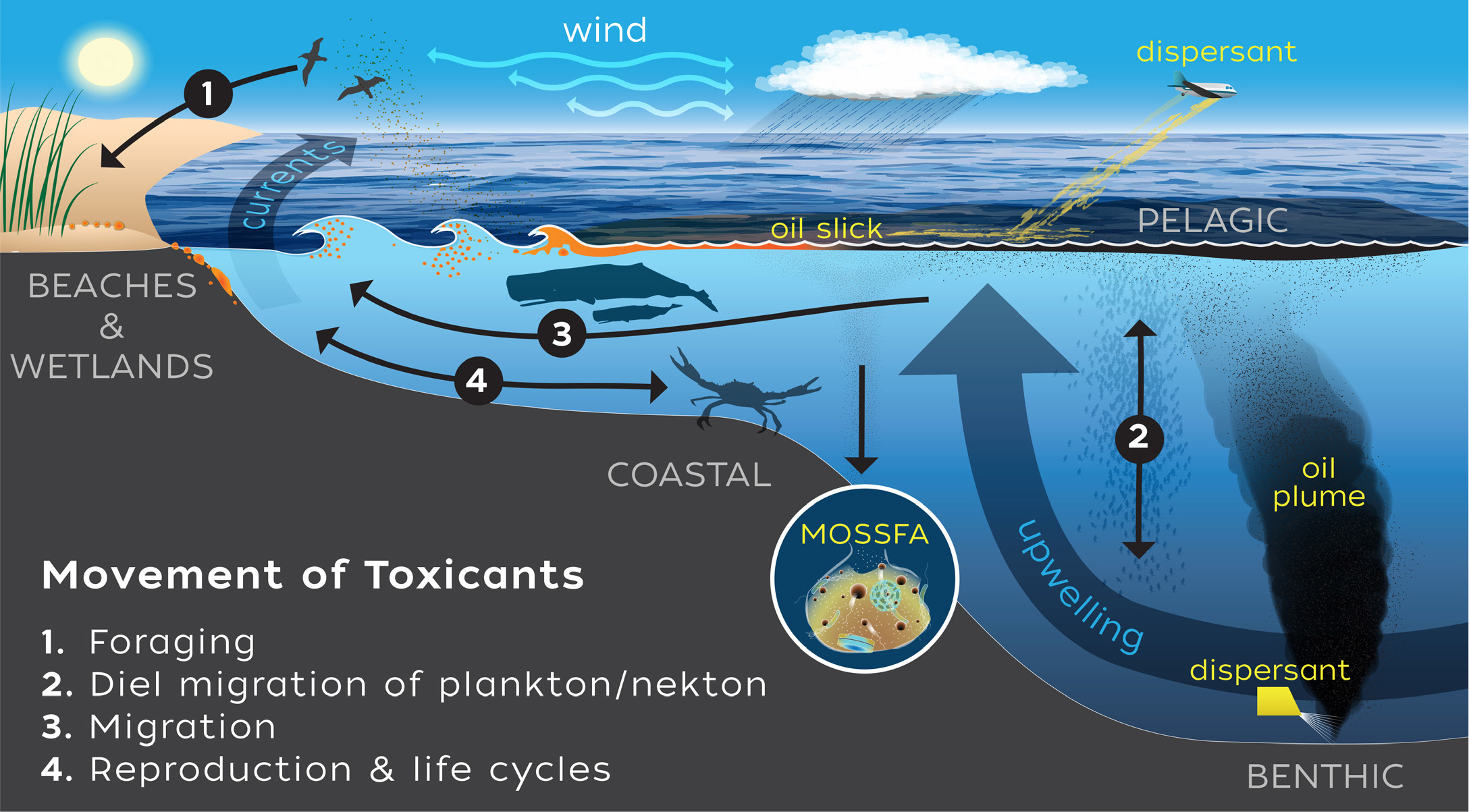
Figure 6. As organisms carrying toxicants enter new areas, they may reproduce, defecate, get eaten, or die and release the byproducts of the Deepwater Horizon spill into novel areas. Movements such as diel vertical migration by plankton or migration for spawning, feeding, or changes in life-history stage move toxicants to new habitats. > High res figure
|
Stable States and Recovery
Moving forward, great concern and effort has been focused on “recovery” of the northern Gulf of Mexico. However, different ecosystems recover at different rates. For example, the nearshore water column has largely recovered to a pre-DWH spill state, but the open ocean mesopelagic water column is still showing major declines in faunal abundance since the spill. Presumably, there are differences in ecosystem function as well, but species interactions and environmental drivers in this ecosystem are poorly understood, making assessment of ecosystem function difficult. Moreover, based on present data, these ecosystems are unlikely to return to a similar state in terms of species composition or function in the foreseeable future.
The deep-sea benthos is also unlikely to quickly return to a pre-DWH state. Schwing et al. (2015, 2020) performed decadal time-series analyses on multiple deep-benthic community size and taxa groups, including benthic foraminifera, meiofauna, and macrofauna. They found that while benthic foraminifera diversity indices and density tended to return to pre-DWH levels within three to five years, statistical analyses demonstrated that the assemblages (species present) prior to DWH were significantly different than those present following DWH, with an increase in infaunal and opportunistic taxa. They reported that an increase in opportunistic taxa was also the case for other meiofauna. However, meiofaunal assemblage changes consistent with DWH impact were superimposed on a longer-term (since the 1980s) decline in ecological quality status.
If the physical substrate of the environment has been altered or if the environment is characterized as low energy (e.g., the deep benthos), recovery to the original state will be improbable or slow. Simply put, if the habitat is no longer available or if there is limited energy for growth and repair, the community in question cannot return to pre-spill conditions. Modeling efforts that seek to incorporate biological information have improved understanding of the potential environmental impacts that may result from given oil spill scenarios (Grüss et al., 2016; Ainsworth et al., 2018; Woodstock et al., 2021). For example, one of the Schwing et al. (2020) studies produced a conceptual model of how organismal interactions in deep benthos environments changed during and following the DWH by including fluxes, increased abundance/activity/respiration, lethal and sublethal effects, and changes in community structure. Moving such models forward will be of considerable importance for identifying gaps in knowledge at all hierarchical levels. Filling these gaps will require basic research.
Even with evidence in hand and the use of predictive models, illuminating drivers that control ecosystem stability and resilience has been exceedingly difficult. One feature common to many Gulf of Mexico systems is the propensity for disturbance and recovery. Perhaps unlike any other region in the world, the Gulf of Mexico is subject to a regular procession of disturbances that include hurricanes, anoxia, and sizable salinity shifts in nearshore environments due to rain or drought. Perhaps the best recent example was the very large freshwater discharge from Hurricane Harvey emanating near Houston, Texas, in 2017. Large plumes of freshwater that lasted for months could be measured well out into the Gulf (Walker et al., 2020). In addition to these factors, several habitats are regularly affected by petrochemicals (e.g., through natural seepage, previous oil spills, and even persistent releases of small amounts of hydrocarbons due to human activities) that also likely impacted the Gulf’s ability to recover from the DWH spill. When considering recovery and restoration, the fact that Gulf of Mexico communities are affected by multiple stressors, from hurricanes to anoxia to the persistent presence of hydrocarbons, should be factored into decisions that will impact the environment.
The presence of multiple stressors has presented challenges for studies in the Gulf of Mexico and can hinder restoration and recovery efforts. Scientists need to be clever in how they tease out the individual effects of multiple stressors to understand their impacts. Multiple stressors do not always work additively; stressors can combine in just the right way to cause tipping points to be exceeded. Lionfish (Pterois volitans) offer an illustrative case as to the difficulty of untangling the difference between causation and correlation, or how much one stressor acted as driver for ecosystem change. Immediately after the DWH spill, there were significant species shifts in northern Gulf of Mexico reef fish communities, with species richness declining 38% and Shannon-Weiner diversity declining 26% (Lewis et al., 2020). At the same time, lionfish were invading the northern Gulf of Mexico region (Kitchens et al., 2017). The environmental insult from the DWH spill may have given lionfish the opportunity to establish themselves and, subsequently, the presence of lionfish may have hindered recovery of native fish fauna. Thus, determining the impact of lingering DWH effects versus the lionfish invasion on reef fishes is challenging.
Although multiple stressors are influencing the Gulf of Mexico, research arising out of GoMRI suggests that prolonged human activities cause the greatest amount of insult to the systems. The DWH spill was a horrendous accident that will impact environments for several decades. By contrast, decades of overfishing, poor land use, and anoxia due to nutrient loading from farming practices have resulted in impacts that will take much longer to correct. In the case of seagrass communities, issues such as trawling by humans, combined with variations in salinity, combined with resuspension of toxic sediments, can make it extremely difficult to determine the impact of any one stressor. After the DWH spill, commercial fisheries were closed from January to April 2011, which included most of the spring reproductive period. These closures were successful in their intended purpose of ensuring public safety and increasing consumer confidence in Gulf of Mexico seafood, but they had little effect on the long-term recovery of fish populations because of the short duration (Ainsworth et al., 2018). Although the surface slick had a brief impact on larval populations (Chancellor, 2015), Atlantis ecosystem modeling suggests that effects on recruitment potential were short-lived and inconsequential compared to longer-lasting toxicological impacts on the breeding population (Ainsworth et al., 2018). Populations often took one or more generations to recover from the acute mortality that resulted from the spill.
What About Next Time?
With >1,000 active and >3,000 total oil and gas rigs sited there, the petroleum industry will continue to have a huge influence on the Gulf of Mexico. The fact that the industry continues to push into deeper waters is a concern, as the DWH spill demonstrated that the flow can be hard to stop in deeper water if safety measures fail. The DWH spill directly impacted over a third of the Gulf of Mexico, but given horizontal transport of species, impacted organisms have moved throughout the Gulf, taking toxicants with them. Decisions made at the time concerning spill mitigation had direct consequences for which environments were the most damaged. Specifically, the decision was made to try and keep as much of the oil as possible offshore and away from marshes, coastal regions, and, importantly, humans. Public perception and financial consequences of this decision should not be overlooked.
Our knowledge of organisms and ecosystem function is vastly different across ecotypes. Few data exist for deep-sea environments because getting appropriate measurements or observations is expensive and technically difficult. Comparatively, inshore and coastal environments are much better understood. This disparity of information has the potential to bias comparative risk analyses and decision-making. A more thorough consideration of management strategies for even routine practices in the oil industry should be explored (Cordes et al., 2016).
Given the likelihood of oil spills in the future, the ability to make informed decisions depends on continued considerable efforts to understand how Gulf of Mexico ecosystems function and how they are interconnected. One particularly difficult research challenge has been discerning the impact dispersants had at the community or ecosystem level. Most knowledge of the biological impacts of dispersants on biota has taken place in laboratory experiments or in controlled mesocosms (Quigg et al., 2021, in this issue). Notably, the chemical cocktails that comprise dispersants can be hard to track in natural environments even when companies are willing to disclose the contents. Dispersants were a component of the marine oil snow event that impacted the deep coral habitats (White et al., 2014). In situ studies have shown that dispersant and oil-dispersant mixtures can have physiological effects that are significantly greater than oil exposure alone (e.g., DeLeo et al., 2016; Ruiz-Ramos et al., 2017), and they can suppress oil biodegradation (Kleindienst et al., 2015). Although the exact cause and mechanism of the injury to the corals may never be known (Fisher et al., 2014), there are specific gene expression patterns of dispersant exposure that may be helpful in determining the direct cause of injury in future spills (DeLeo et al., 2018).
Moving forward, there is an opportunity to vastly improve our ability to understand and possibly mitigate oil spills (and other disasters). We present four areas of research that are particularly critical for improving understanding.
1. Establishing monitoring systems that provide essential environmental and ecosystem data. Pre-DWH spill data were very limited for some environments, making assessments of damage and ecosystem impacts difficult. Looking forward, monitoring systems will provide baseline information that facilitates assessment of the next environmental insult, be it an oil spill, hurricane, or something different. Retrospectively, monitoring is needed to help understand the long-term recovery in the wake of the DWH, especially for mesopelagic and deep-sea environments where ecosystem processes run more slowly.
2. Quantifying magnitudes and rates of exchange (i.e., coupling) between Gulf of Mexico ecosystems. We have little understanding about the rates, magnitudes, or in some cases mechanisms of processes that allow transport within and across Gulf of Mexico ecosystems. Examining, for example, benthic-pelagic coupling, mesopelagic to epipelagic coupling, and nearshore to salt marsh coupling would allow researchers to understand how toxicants can remain active and move between environments.
3. Developing criteria for assessing the “vulnerability” and “resilience” of species, communities, and ecosystems. For many organisms, we have a very poor understanding of their vulnerability to insult or injury (Murawski et al., 2021, in this issue); this is especially true for open ocean or deep-sea animals (Schwing et al., 2020). Likewise, in many cases we do not know which species are critical for maintaining ecosystem function. Criteria for defining vulnerability and resilience will vary depending upon species, community, and ecosystem and will help build more robust working parameters.
4. Developing predictive modeling that incorporates biological and physical processes. Just as biophysical models were used to predict where and when oil might disperse (Paris et al., 2012), integrative models that forecast how biological systems might behave, or what functions of the ecosystem are particularly vulnerable, would be invaluable to both scientists and resource managers.
These four areas of development are broadly applicable not just for understanding oil spills in the Gulf of Mexico but are relevant to understanding and mitigating any disaster.
Conflict of Interest
The authors declare that the research was conducted in the absence of any commercial or financial relationships that could be construed as a potential conflict of interest.
Author Contributions
All authors contributed to the analysis, evaluation, and writing leading to this contribution. Funding for the project was primarily provided by the Gulf of Mexico Research Initiative.
Acknowledgments
In particular, we thank the Gulf of Mexico Research Initiative Core Area 3 “motivators” (including those not listed as authors here: Margaret Leinen, Bill Hogarth, Bob Shipp, John Farrington, and John Shepherd) for their help and encouragement. A special thanks goes to Chuck Wilson for his tireless efforts to make GoMRI science the best it can be, including helpful review of and additions to this manuscript. In addition, we thank the staffs of the Consortium for Ocean Leadership and the American Institute of Biological Sciences for travel and logistical support, including Michael Feldman, Callan Yanoff, and Jennifer Petitt. Additionally, Natalie Renier at WHOI Graphic Services was most helpful.