The Loop Current and Productivity Along the West Florida Shelf
Often considered to be oligotrophic, the west Florida continental shelf (WFS) supports abundant fisheries, and at times it is beset by copious quantities of the harmful alga Karenia brevis, raising the question of how it is possible for an oligotrophic shelf to be so productive. The answer is that the WFS is not always oligotrophic. To understand why this is so and to appreciate the ecological consequences, we must consider how the WFS is forced, in particular, by the adjacent western boundary current—the Gulf of Mexico Loop Current (LC).
The first consideration is the geometry of the eastern Gulf of Mexico (Figure 1), where we observe that the gently sloping WFS is as wide as the dry land mass of the Florida peninsula. The LC abuts the WFS slope region at times, entering the Gulf of Mexico through the Yucatán Strait and exiting through the Straits of Florida as the Florida Current and the Gulf Stream. While in the Gulf of Mexico, the Loop Current/Florida Current/Gulf Stream system penetrates northward before looping around to exit, occasionally shedding a large anticyclonic eddy and retracting back to the south. Within its penetrating, eddy-shedding, and retraction evolutionary process (e.g., Nickerson et al., 2022), the LC often interacts with the WFS slope at various locations. Figure 1 includes an example of such eddy shedding.
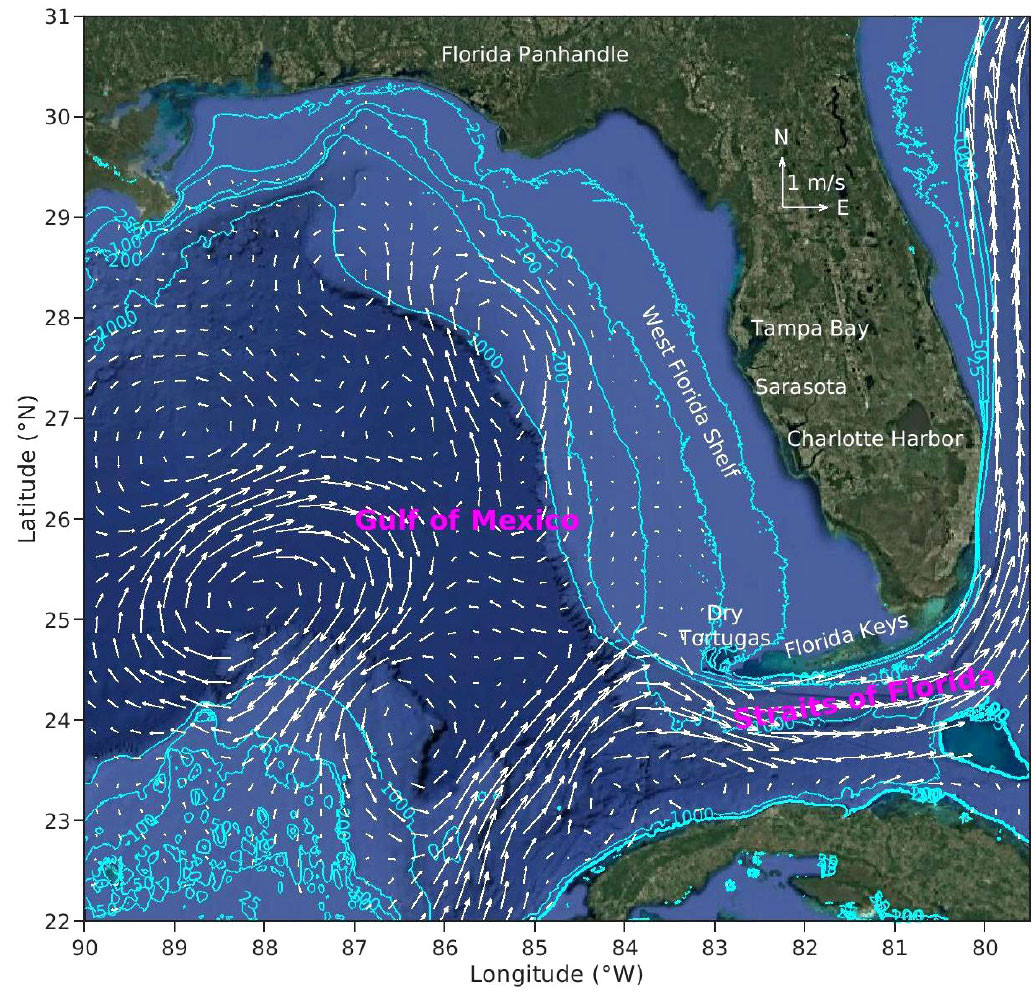
FIGURE 1. The configuration of the Loop Current system within the Gulf of Mexico, as estimated for September 15, 2018, using AVISO satellite altimetry. The arrows denote the surface geostrophic currents in m s–1 calculated from the height gradients. The contours are the 25 m, 50 m, 100 m, 200 m, and 1,000 m isobaths. Regions of discussion are labeled. Note the separation of an anticyclonic eddy from the parent Loop Current and the subsequent contact by the Loop Current with the southwest corner of the west Florida shelf near the Dry Tortugas, the region referred to as the pressure point. > High res figure
|
Geophysical fluid dynamical constraints are such that a sea surface height displacement (or pressure perturbation) imposed upon the shelf slope can only penetrate landward by a distance equal to a Rossby radius of deformation (about 30 km for the WFS, given the local Coriolis parameter and water properties) and that such a perturbation may also propagate with shallow water to its right (i.e., northward along the WSF slope). Thus, LC effects on the WFS are limited to the outer shelf except when the LC impacts the shelf slope at its southernmost extent near the Dry Tortugas. Because the Dry Tortugas are the westernmost islets in the Florida Keys chain, all isobaths of about 20 m and deeper must wrap around the Dry Tortugas. Hence, if the LC contacts the shelf slope near the Dry Tortugas, its impact (by contacting shallow isobaths within a Rossby radius of deformation) can extend across the entire WFS, thereby setting up a shelf-wide upwelling circulation. This circulation enables upper shelf slope water (with elevated inorganic nutrient concentrations) to flow over the shelf break and transit across the shelf toward the shore within the bottom Ekman layer. The companion works of Weisberg and He (2003) and Walsh et al. (2003) demonstrate this occurrence and its effect on phytoplankton ecology.
K. Brevis Bloom Prediction
Subsequent analyses by Liu et al. (2016), using a growing set of observations (K. brevis cell counts, LC position via satellite altimetry, long-term velocity and water property observations from moorings, plus glider and shipboard transects), led to the development of a seasonal prediction scheme regarding whether a major K. brevis bloom would materialize along the WFS in any given year. K. brevis is a slow growing dinoflagellate that can only outcompete faster growing diatoms under oligotrophic conditions, which in most years are limited to the mid-shelf, away from either nutrient inputs by land drainage, as occurs nearshore, or deeper-ocean nutrient inputs, as occurs at the outer shelf. This mid-shelf, oligotrophic scenario changes in years when the LC makes protracted contact with the shelf slope near the Dry Tortugas, a location we refer to as the WFS pressure point. If this occurs prior to the spring to summer phytoplankton bloom period, then diatoms are favored over K. brevis, negating a nearly annual occurrence of such a harmful algae bloom. Exceptions do occur to this simple scenario; nonetheless, for the years subsequent to 1993, when joint cell count and satellite altimetry observations exist, the simplistic pressure point hypothesis prediction score card has a winning record.
K. brevis blooms do not depend only on mid-shelf nutrient conditions; they also require a delivery mechanism from the mid-shelf to the nearshore to become a nuisance bloom. Transport within the bottom Ekman layer under upwelling conditions was confirmed via observations for the 2012 bloom event (Weisberg et al., 2016). Combined, the nutrient and delivery mechanisms result in considerable interannual variations in nearshore red tide bloom onsets, intensities, and durations. Some years are truly disruptive, whereas others are not. The K. brevis bloom of 2018 provides a case study for which cell count and glider and moored instrumentation observations, coordinated with numerical circulation model simulations, demonstrate locations, sequencing of intensities, and eventual abatement of what was arguably the worst of the red tide blooms to affect Florida in recent decades.
2018 K. Brevis Bloom
As described in Weisberg et al. (2019), the LC remained in a penetrative state without any WFS slope contact until around mid-July of 2018. This changed once the LC shed an eddy, allowing the LC to sidle eastward to interact with the pressure point, an interaction that lasted through the end of December 2018. Figure 1 shows the LC configuration for September 15, 2018. A glider survey conducted from August 24 through September 17, 2018, documented the water properties of the mid-WFS, identifying near-bottom, upwelled water with high chlorophyll (identified as being K. brevis related) located roughly between the 30 m to 40 m isobaths. A subsequent numerical circulation model simulation (employing the West Florida Coastal Ocean Model, an adaptation of the Finite Volume Community Ocean Model of Chen et al. [2003] nested in the HYbrid Coordinate Ocean Model of Chassignet et al. [2009]), with neutrally buoyant particles initialized along the glider track, then demonstrated the fluid pathways for the glider sampled water parcels to the nearshore (Figure 2). This helped to explain (1) an elevation in cell concentration from what had been a lingering 2017 bloom (Figure 3) after the LC made its pressure point contact, and (2) a substantial increase in bloom intensity in the Tampa Bay region. These transport findings were also consistent with the timing of K. brevis cells appearing off Florida’s panhandle and east coasts, as explained in Weisberg et al. (2019).
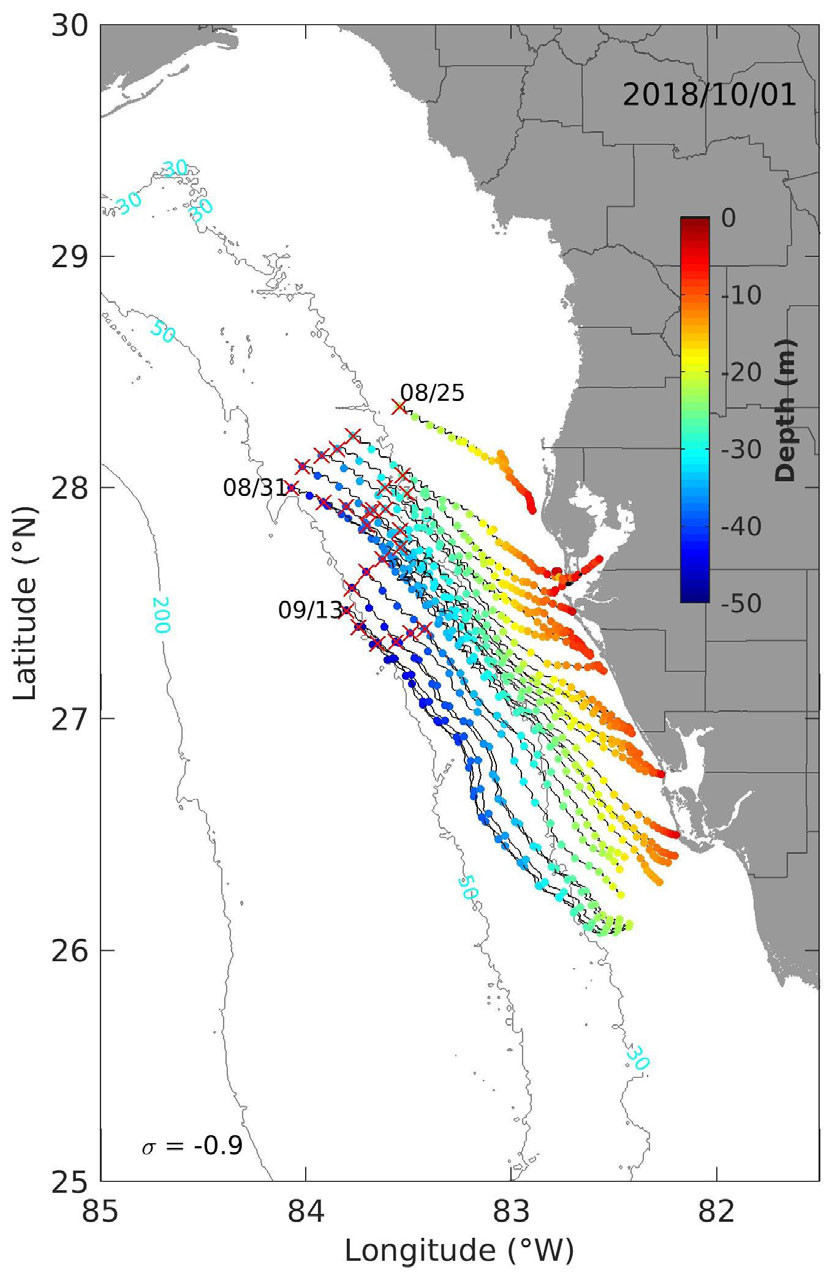
|
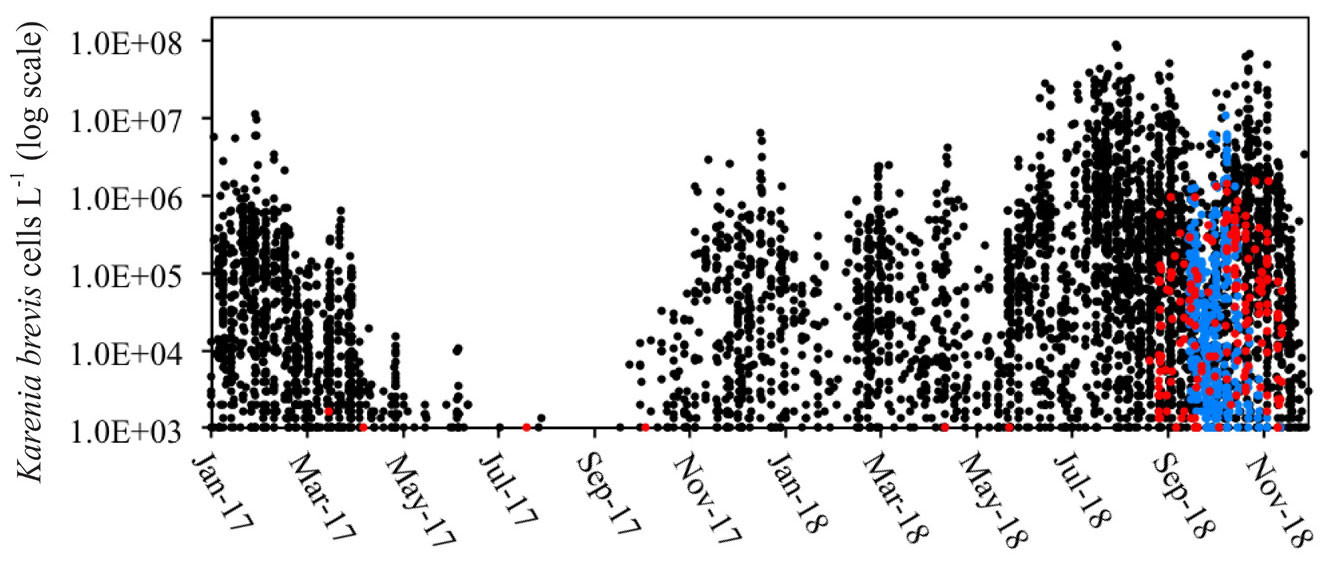
FIGURE 3. K. brevis cell counts, plotted using a log scale from January 1, 2017, through December 16, 2018, show the persistence of the K. brevis bloom from 2017 through the spring 2018, the increase in intensity in the central west Florida shelf epicenter region in summer 2018 (black dots), and the durations of bloom conditions on the Florida panhandle coast (red dots) and on the Florida east coast (blue). Only samples with ≥103 cells per liter are shown. Data source: Florida Fish and Wildlife Conservation Commission - Fish and Wildlife Research Institute HAB monitoring database. Figure from Weisberg et al. (2019). > High res figure
|
Given such a nexus between the observed, mid-shelf, near-bottom formation region and the subsequent delivery to the nearshore, is it possible to employ the same coastal ocean circulation argument to account for the eventual cessation of such a K. brevis bloom? To address this question, Liu et al. (2022) posed the following: How long might it take for a continuing upwelling circulation to flush the nearshore situated cells back out to sea once an offshore source is depleted? This is a physically reasonable question to ask because we know from Weisberg et al. (2019) that nearshore cells were transported offshore to eventually be entrained into the LC and thus transported to Florida’s east coast. A numerical experiment was performed by initializing a normalized tracer concentration (equal to 1.0) at all of the model grid cells (surface to bottom) located inshore of the 10 m isobath from just north of Tampa Bay to Naples, Florida, and then running the model with available local forcing information, including winds, heat fluxes, and river inflows, plus deeper-ocean forcing by LC interaction at the pressure point and elsewhere along the shelf slope. The tracer concentration as a function of time was then compared with the observed cell concentration, with the results showing a qualitative consistency with the observed K. brevis cell concentrations (Figure 4).
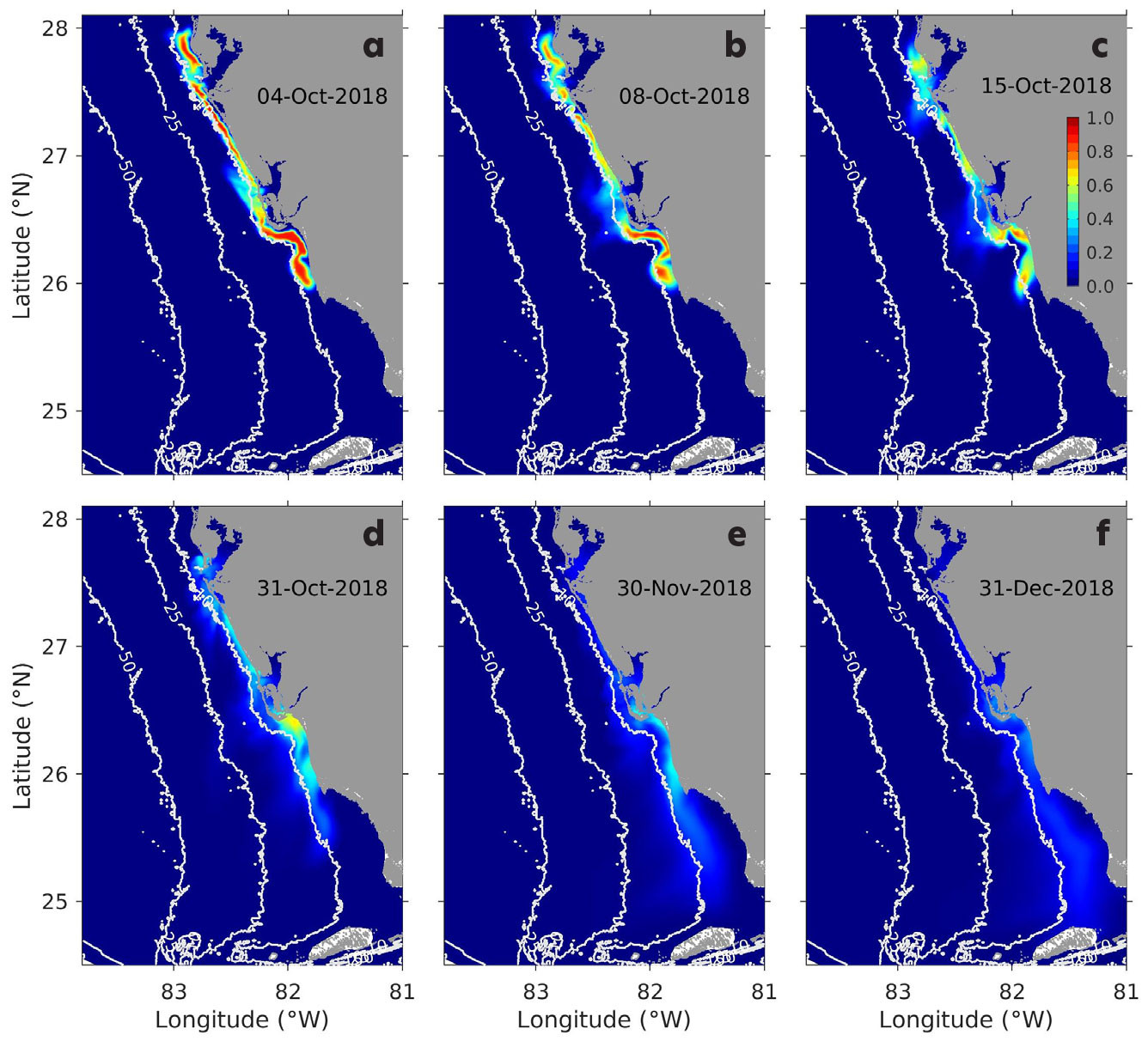
FIGURE 4. Snapshots of model-simulated surface tracer concentrations for the central to southern west Florida shelf relative to the 10 m, 25 m, and 50 m isobaths. The snapshots are sampled after the initial release at (a) three days, (b and c) one and two weeks, respectively, and (d–f) one, two and three months, respectively. The color-coding shows the decrease in tracer concentration. Adapted from Liu et al. (2022). > High res figure
|
Broader Implications
While our discussions here focus on the WFS, our findings have broader implications. Whereas all continental shelves respond to both local and deeper-ocean forcing influences, their individual geometries may make these influences less discernible than those for the WFS. For instance, the WFS width enables us to distinguish between inner-shelf and outer-shelf regions and hence the relative imprints imposed by local and deeper-ocean forcings. Additionally, regions like the Dry Tortugas, where shallow isobaths are approachable by adjacent boundary currents, are not limited to the WFS. Cape Hatteras presents an opportunity for the Gulf Stream to impact the entire South Atlantic Bight, and similar situations may occur wherever boundary currents and their associated eddies may come into contact with wide continental shelves at locations where the shelf may narrow down to a Rossby radius of deformation.
Conclusion
It would be an oversimplification to say that ocean circulation physics fully controls K. brevis bloom evolution, but it is fair to say that ocean circulation physics are on an equal footing with the organism’s biology in determining K. brevis bloom inception and duration. Subsequent experiments with biological elements added to the otherwise purely physical transport mechanism are yielding modifications, but none that would negate the basic finding that by determining the water properties in which K. brevis may, or may not, thrive, plus the mechanism by which K. brevis may be transported, the ocean circulation forms an integral part of interdisciplinary harmful algal bloom studies.
What applies to harmful algae should also apply more generally to all matters of coastal ocean ecology. By recognizing that the coastal ocean (whether at the WFS or elsewhere) is driven by a combination of local and deeper ocean forcing factors and that to study these requires coordination between both long-term observations and model simulations, we will collectively continue to advance our knowledge of what controls this essential region where society meets the sea.
Acknowledgments
Current support for this work is by the Southeast Coastal Ocean Observing Regional Association (SECOORA) as a pass-through from NOAA IOOS (award #NA21NOS0120097), a cooperative agreement between NOAA’s Office of Coast Survey and the University of South Florida through the Center for Ocean Mapping and Innovative Technologies (COMIT, award #NA20NOS4000227), NOAA National Centers for Coastal Ocean Science Competitive Research Program (award #NA19NOS4780183) for which this is ECOHAB contribution #1114, and the State of Florida through FWC/FWRI (agreement #20035).