INTRODUCTION
Offshore wind power, now in the nascent stages of development in the United States, is poised to grow rapidly over the next decade (Bowers, 2020) toward a capacity over 2,000 gigawatts—more than double the generating capacity of existing US electric power plants (US DOE, 2020; Figure 1). Currently, two projects are operating in US waters—Block Island Wind Farm (BIWF) off the coast of Rhode Island (operated by the Danish power company Ørsted) and Coastal Virginia Offshore Wind (US company Dominion Energy)—totaling 42 megawatts of capacity. Utility-scale projects contributing nearly 24 gigawatts of generation are in the planning and/or permitting stages off the US eastern, Great Lakes, and Pacific coasts (Figure 2). The first project—Vineyard Wind (Massachusetts)—is in its final federal regulatory review process, with federal approval anticipated in late 2020.
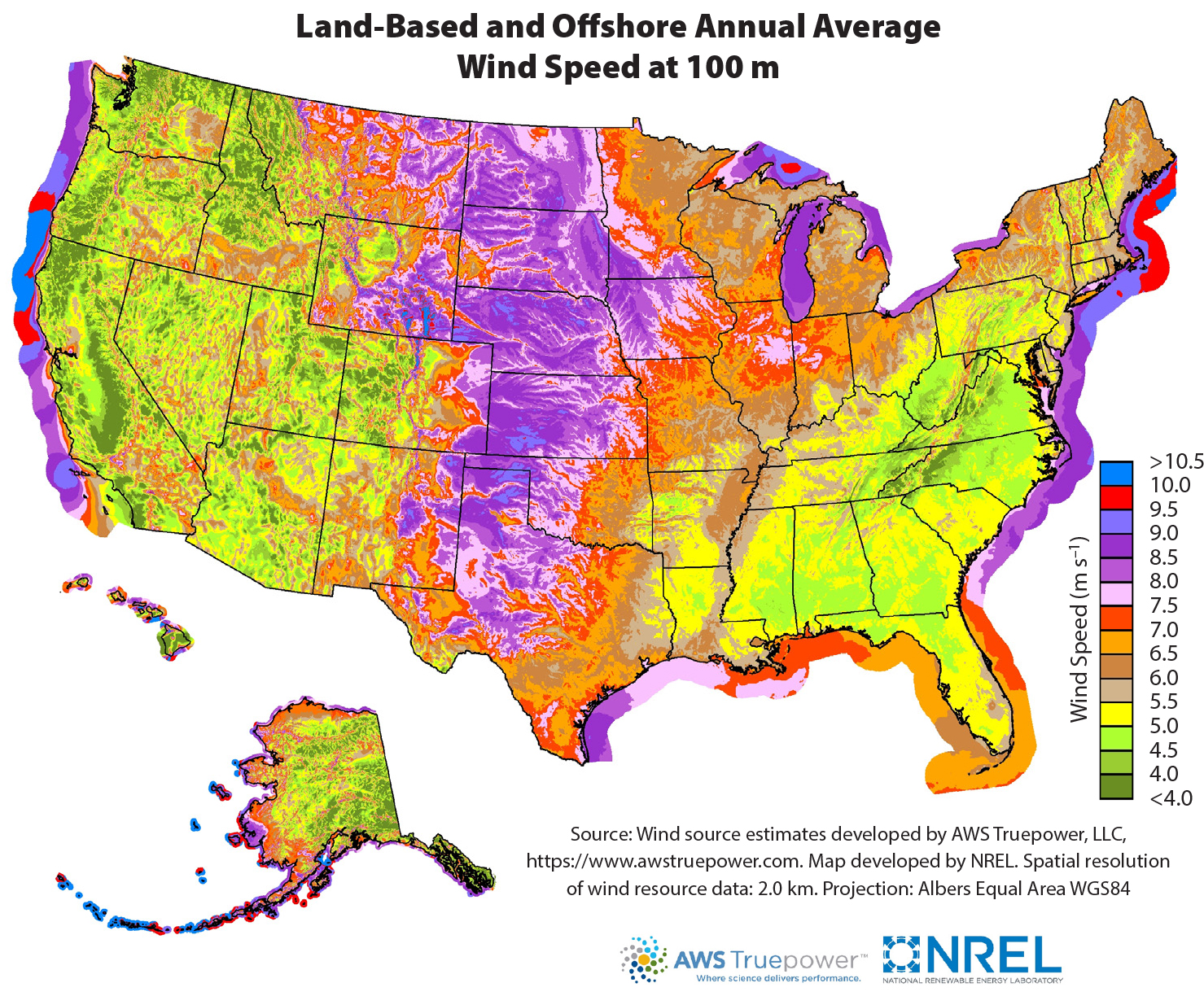
Figure 1. US seaboard wind resource potential is strongest along the Northeast, Mid-Atlantic, and California coasts, indicated by the blue, red, and purple shading, as modeled based on average annual wind speeds by the National Renewable Energy Laboratory (https://www.nrel.gov/gis/wind.html). > High res figure
|
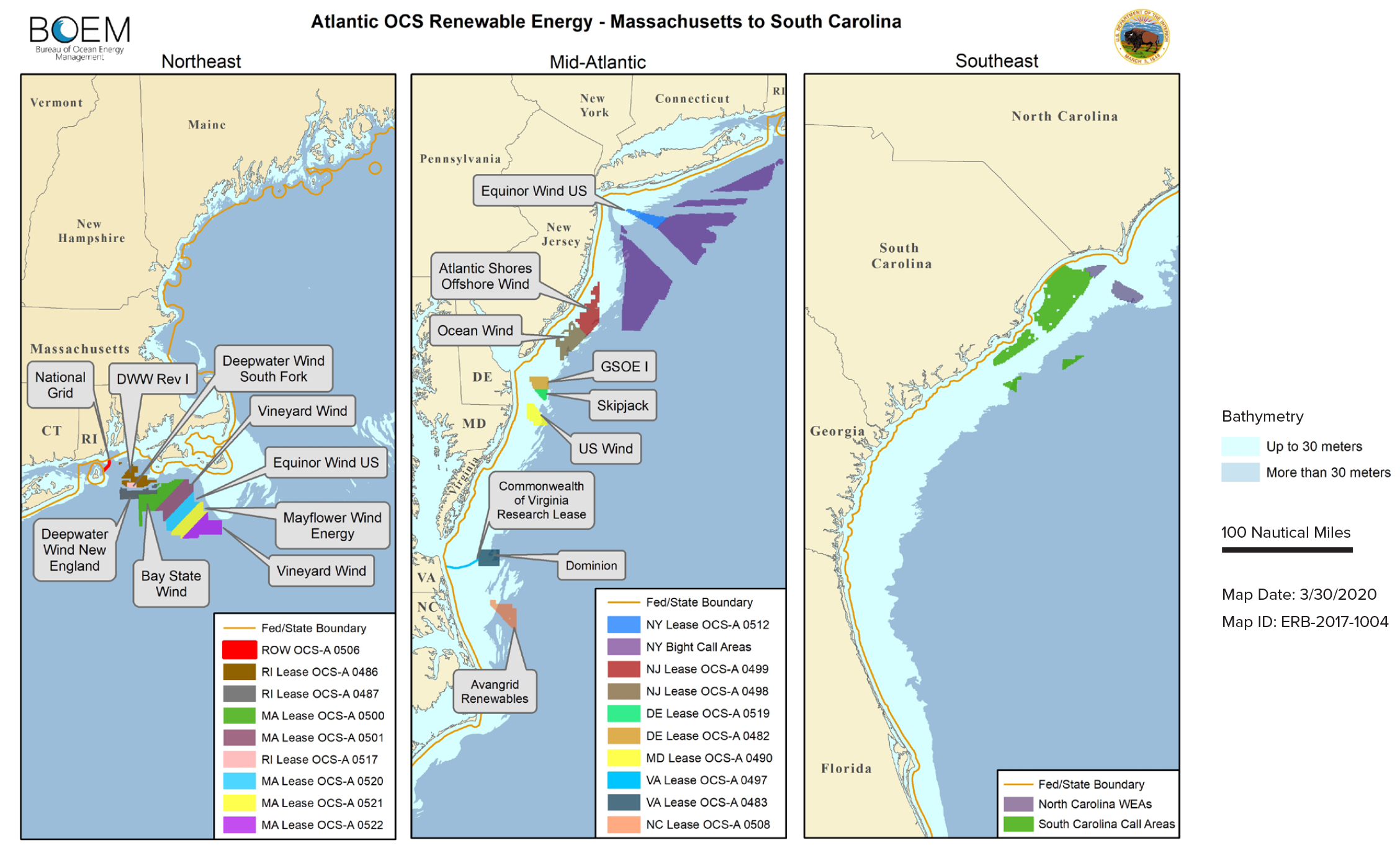
|
Approximately 56 million people live in mid-Atlantic and northeastern states, and nearly 80% of them live in coastal regions (US Census Bureau, 2019). As a function of increasing population density and energy costs, these regions have the highest demand for electricity in the United States. In response, state governments are working to meet the needs of their coastal populations and reduce their carbon footprints by creating legislation to accelerate the procurement of renewable generated power, with examples including the state of New York targeting 9,000 megawatts for development by 2035 and the state of Massachusetts intending to purchase 3,200 megawatts by 2035 (AWEA, 2020). Offshore wind will be a significant contributor to the American economy. Recent data show the upward economic trajectory, with predictions for nearly $78 billion in capital spending to occur this decade (Cohen, 2020).
However, East Coast commercial and recreational fishing industries are equally important. The US Atlantic, where wind leasing is concentrated, has a long and strong tradition and reliance on fishing. Domestic fisheries contribute about $2.5 billion to the Mid-Atlantic region’s economy (MAFMC, 2020). In New England, the landings and revenue generated are nearly three times greater (NMFS, 2018). In Massachusetts, where the first large-scale offshore wind projects will be built, commercial fishing was one of the state’s first maritime industries and now supports approximately 87,000 jobs and is home to top port by value (New Bedford, $431 million) for the nineteenth consecutive year (NMFS, 2018). The needs of each fishing group vary depending on the species targeted, habitat type, season, type of gear used, and methods for deployment. The diversity in fish species and fishing activities presents challenges and opportunities for considering how to evaluate impacts of offshore wind farms before the first large-scale projects are constructed (NMFS, 2019).
Monitoring and research are critical for evaluating fisheries impacts from each project as well as cumulatively along the US Atlantic seaboard. Thus, the intent of this paper is to provide a brief perspective on the current knowledge available from offshore energy industries abroad and in the United States and to highlight initial studies where data will be made available in the next one to three years. We will also discuss how, if coordinated and done in partnership, these efforts can support an early process for the adaptive management of wind impacts on fisheries and fishing.
REGULATORY FRAMEWORKS
The US offshore wind regulatory landscape is multidimensional. Projects will generate energy in US federal waters, and that energy will be delivered to onshore facilities located on state, local, and private lands for distribution by state utility grids. Governing statutes, regulations, and policies at federal, state, and local levels require a high degree of coordination. Leasing and development of offshore energy and mineral resources is governed by the Outer Continental Shelf Lands Act (OCSLA) of 1953 (43 US Code § 1332(3)). An amendment to OCSLA—the Energy Policy Act of 2005—designated the Bureau of Ocean Energy Management (BOEM) as the lead authority for issuance of offshore renewable leases, easements, and rights of way (BOEM, 2016). Under this Act, BOEM has developed best management practices, including how projects should work with fishers (BOEM, 2014) and what project-area data will be collected. As required by the National Environmental Policy Act of 1969 (42 US Code § 4321-4347), data collected by BOEM and consulting agencies (e.g., NOAA) are used to assess a project’s potential direct and cumulative environmental effects and impacts. While BOEM is the lead authority for issuance of federal offshore wind projects, the National Marine Fisheries Service (NMFS), a part of NOAA, is the federal agency responsible for management and conservation of fisheries (Magnuson-Stevens Fishery Conservation and Management Reauthorization Act of 2006, Public Law 109-479, 121 Stat. 3575 (2006)). In regard to impacts on fisheries and fishing, the divisions of authority require close coordination and consultation between federal and state agencies and the developers.
OFFSHORE WIND WILL CREATE CHANGE
Offshore wind development will further change marine systems that are already undergoing natural and climate-induced changes (Saba et al., 2015). Prediction and estimation of fisheries impacts are particularly difficult in areas where the installations are novel and utility-scale (e.g., East Coast wind farms with hundreds of new structures expected in the coming decade) and where impacts may be of long duration (30 plus years).
Fisheries resources and habitats are subject to natural and anthropogenic stressors (Saba et al., 2015), including warming temperatures from climate change (Henson et al., 2017), ocean acidification (Kroeker et al., 2013), and ocean deoxygenation (Oschlies et al., 2018). Rapid climate-induced shifts are affecting habitats and species distributions in proposed wind development areas (Torre et al., 2018). Nye et al. (2009) evaluated changes in the distributions of 36 fish stocks in northeast US continental shelf waters between 1968 and 2007 and showed that the biomass of many of these stocks shifted toward the north and expanded into deeper habitats and cooler waters in response to climate-driven environmental changes. Given the uncertainty caused by these climate-induced factors, assessments of wind development impacts on fisheries must anticipate, account for, and try to measure the complexities of associated changes in species distributions (Pinsky et al., 2018).
Offshore wind project impacts are assessed by phases (construction, operations, and decommissioning) under the US regulatory framework (BOEM, 2019). Impact-producing factors (IPFs) range from cable disturbances to turbulence generated by the structures (BOEM, 2019). Studies to investigate these IPFs started with the Block Island Wind Farm (BOEM, 2020a). Acknowledging there are many IPFs, here we focus only on habitat creation and reef effects resulting from the presence of wind structures, specifically turbine foundations and scour material (typically rubble and coarse gravel).
EUROPEAN CASE STUDIES
European offshore wind studies published between 2010 and 2020 can be used as proxies for reef effect impacts on analogous species. The European ecological and social studies can also inform US efforts regarding potential impacts of foundations on fish, shellfish, and the broader marine environment (Langhamer, 2012). Many of these studies are discussed in greater detail in this special issue. Here, we summarize key findings that may correlate to analogous species present in US Atlantic wind energy areas.
A 10-year research program conducted in Germany provides a multiyear perspective (Lüdeke, 2015) and a synopsis of wind effects on a broad range of marine resources. Overall, the results could not confirm negative effects on benthos or fish from the presence of turbines. A second pre-construction and operation study, lasting seven years, showed an increased number of species during the operation phase along with greater total fish biomass and increased biomass of shore crab (Carcinus maenas; Langhamer et al., 2016) and cod (Gadus morhua; Bergstrom et al., 2013).
European offshore turbines have also been shown to influence attraction, avoidance, and site fidelity of certain fish species and their prey. Differences in fish densities measured between foundations extending through the entire water column and the surrounding ocean waters showed a positive effect around the foundations (Andersson and Öhman, 2010). Research has indicated increased fish assemblages (Wilhelmsson et al., 2006) over both short (Lindeboom et al., 2011) and long terms (Stenberg et al., 2015), and has provided observations of increased biodiversity and biomass of certain crabs (Cancer pagurus; Krone et al., 2017) and lobster (Homarus gammarus; Roach et al., 2018) on hard substrate habitat created by turbines and associated scour protection. Both the hard substrate of the steel pilings and the scour protection created new habitats and shelters that were immediately colonized by marine species. Reubens et al. (2011) showed that the type of epifaunal food resources colonizing wind structures influences fish communities, with species selecting prey that live specifically on the structures. Seasonal attraction and site fidelity have also been documented for age groups of cod and pout (Zoarces americanus) at wind farms (Reubens et al., 2014). While attraction has been documented for certain species (cod, crab [Necora puber and Cancer pagurus], lobster), observations also indicate avoidance by other species, such as flatfish (Limanda limanda, Solea solea, and Buglossidium luteum) and whiting (Merlanguis merlangus), but such differences could not be discerned between the physical structures and the impacts that season and weather conditions also have on fish presence (Van Hal et al., 2017).
REEF EFFECTS OF US OIL AND GAS PLATFORMS
Many studies done around the world illustrate the potential value of offshore platforms of all types for enhancing local fisheries. As free-standing hard substrate, it is widely recognized across the US scientific community that oil and gas platforms in subtropical (Gulf of Mexico; Ajemian et al., 2015; Cowan and Rose, 2016) and temperate (California; Claisse et al., 2014) waters serve as artificial reefs, benefiting fisheries from nearly the moment they are installed to decommissioning (Figure 3). Platforms provide habitat for settlement and recruitment of marine invertebrates, encrusting algae, and other sessile organisms that collectively comprise the “biofouling” community (Steimle et al. 2002; Kolian et al., 2017). Colonizing organisms in turn establish a food source for higher trophic levels (Raoux et al., 2017). Platforms provide essential fish habitat (e.g., breeding, feeding, recruitment, shelter) for diverse assemblages of large pelagic and demersal fish (Love et al., 2012; Krone et al., 2017). In the Gulf of Mexico, platforms furnish local and regional benefits to highly migratory species (Snodgrass et al., 2020) and serve as spawning habitats for other types of fish (Heyman et al., 2019). In some instances, platforms expanded particular fish populations in an otherwise habitat-limited bottleneck (i.e., red snapper Lutjanus campachanus; Gallaway et al., 2009), or result in some of the highest fish densities (per area of sea bottom) compared to any other habitat on Earth (Claisse et al., 2014). Structures can also play an important role in enhancing connectivity for both native (Love et al., 2012) and non-native invasive (Viola et al., 2018) species. This role may be particularly important as species’ distributional ranges are in flux (Pinsky et al., 2018) and will need to be considered early.
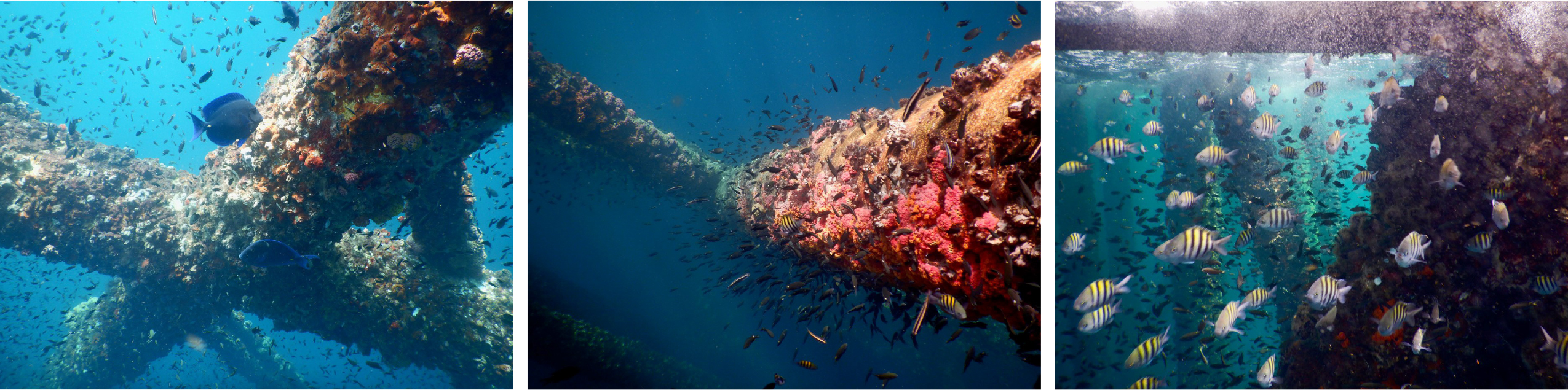
Figure 3. Offshore oil and gas platforms provide hard substrate for biofouling communities and critical habitat for grazers and predatory fishes. Invasive species, for example, Tubastrea sp. can also colonize structures as shown in the central image. From LGL (2020) > High res figure
|
CONSIDERATIONS FOR REEF EFFECTS
European and US experiences indicate a critical need for focused research to evaluate reef effects of installed, and eventually decommissioned, offshore wind foundations and to establish the appropriate baseline monitoring techniques for comparative studies of biomass and density changes, attraction versus production of species, and ecosystem trophic shifts. Fisheries managers and scientists will need robust methodologies to identify and decipher how wind installations impact fish species and stocks. Designing project-specific studies of connectivity and species distribution will also be important, given drivers such as increasing sea surface temperatures that are already impacting fisheries in the US Atlantic region. Evolving offshore wind foundations (i.e., floating structures in deeper waters) will also have to be considered for their unique reef effects. Project-specific and regional monitoring programs in which the data are standardized to compare against federal stock assessments (NOAA Fisheries, 2020) will be required to inform cumulative impacts as US offshore wind development expands.
RECENTLY INITIATED PROGRAMS
Significant efforts are underway to characterize and summarize species baseline distribution and abundance within US offshore wind lease areas. These efforts, required by federal and state regulations, are initial steps for informing and documenting impact and/or change in fisheries and their habitats in and around these areas. Here, we highlight a few of the developer-initiated and government projects not addressed in the other publications in this special issue that will contribute to US regional coordination efforts currently underway.
Federal and State Agency Programs
With rapidly increasing state procurement targets (New York announcing 9 gigawatts by 2035) and 15 project development plans to be submitted over the next two years (BOEM, 2020b), federal and state agencies are funding efforts to collect fisheries data, for example, the Real-time Opportunity for Development Environmental Observations (RODEO) at Block Island Wind Farm (BOEM, 2020c). Table 1 summarizes key federal and state efforts and highlights states’ efforts initiated in the last year that will produce data and results over the next one to two years.
Table 1. Recently funded government programs and studies supporting the coexistence of offshore wind and fisheries along the US Atlantic coast. > High res table
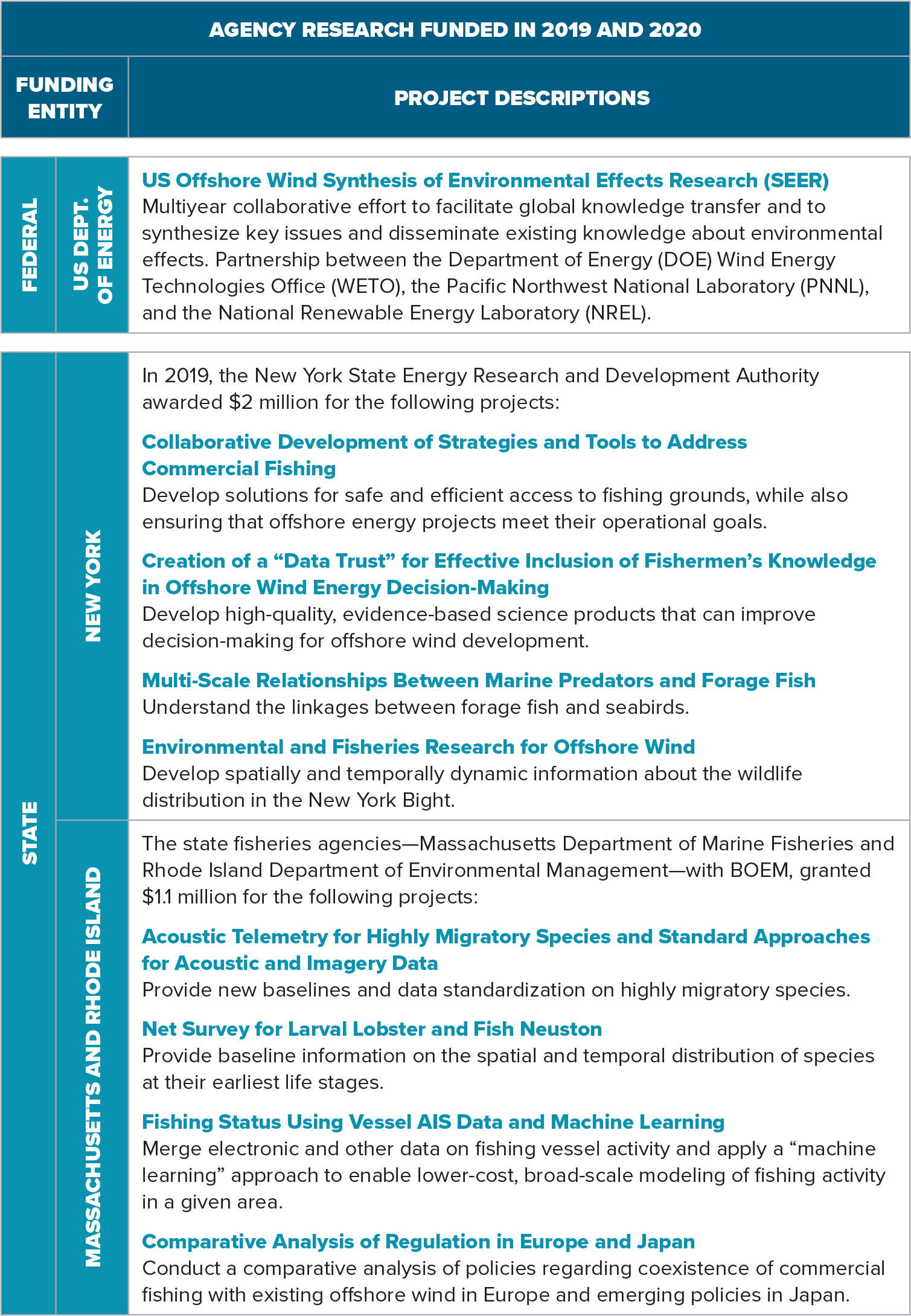
|
Wind Developer Programs
Wind developers are initiating project baseline surveys and planning for pre-construction fisheries surveys for individual projects along the US Atlantic coast. The furthest along in this process is Vineyard Wind, which is (1) surveying and tagging the American lobster (Homarus americanus) and the black sea bass (Centropristis striata), (2) undertaking a drop camera survey, and (3) conducting a demersal trawl survey.
The School for Marine Science & Technology (SMAST), University of Massachusetts, Dartmouth, is using a combination of methods (video and trawl sampling) with before-after-control-impact (BACI) designs is being used to assess populations, community structures, abundances, and/or ages of individual species (SMAST, 2020; Figure 4).
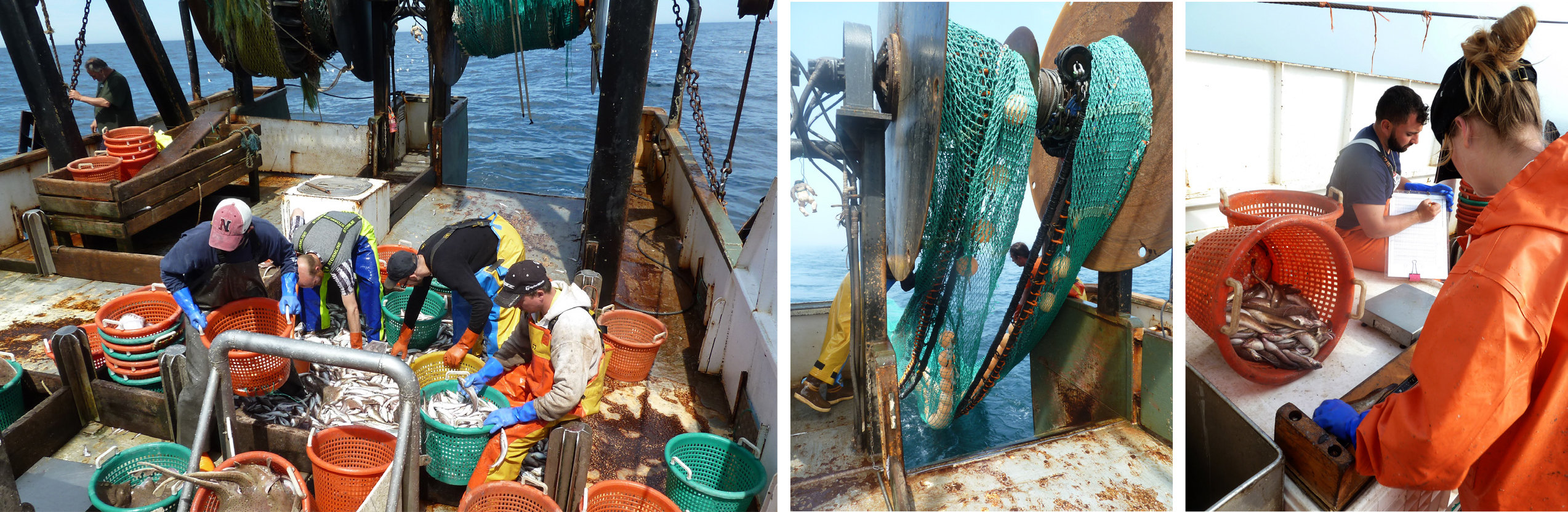
Figure 4. Field images taken during SMAST trawl surveys conducted in the Vineyard Wind project area. Courtesy of Vineyard Wind LLC and University of Massachusetts Dartmouth School for Marine Science & Technology. > High res figure
|
Recreational fishing effort studies for highly migratory species (HMS) in the southern New England Wind Energy Area are also underway (Kneebone and Capizzano, 2020). These studies combine fisheries-dependent interpolated data, fishing effort derived from previous HMS surveys, and conventional tagging data to identify HMS activity in the Wind Energy Area. In the near future, this work will expand to provide a higher resolution view of HMS stocks and recreational effort for all proposed projects in the US Northeast and the Mid-Atlantic.
To meet regulatory requirements, wind developers are deploying one or more standard oceanographic buoys with light detection and ranging (lidar) capability to assess wind resources in and around their lease areas. To promote data transparency, developers are providing access to the data through existing NOAA Integrated Ocean Observing Regional Associations portals: the Northeast Regional Association of Coastal Ocean Observing Systems (NERACOOS, http://www.neracoos.org/realtime_map) and the Mid-Atlantic Regional Association Coastal Ocean Observing System (MARACOOS, https://oceansmap.maracoos.org/; Figure 5). Atlantic Shores Offshore Wind LLC, Mayflower Wind Energy LLC, and Ørsted were the first to provide data that can be easily accessed by fishers and scientists (Table 2). Mayflower Wind Energy LLC is also supporting the extension of the geographic range for an existing study by adding an acoustic device to monitor tagged cod crossing into its lease area. Data collected will help map cod spawning habitat in southern New England (Massachusetts Division of Marine Fisheries, 2020).
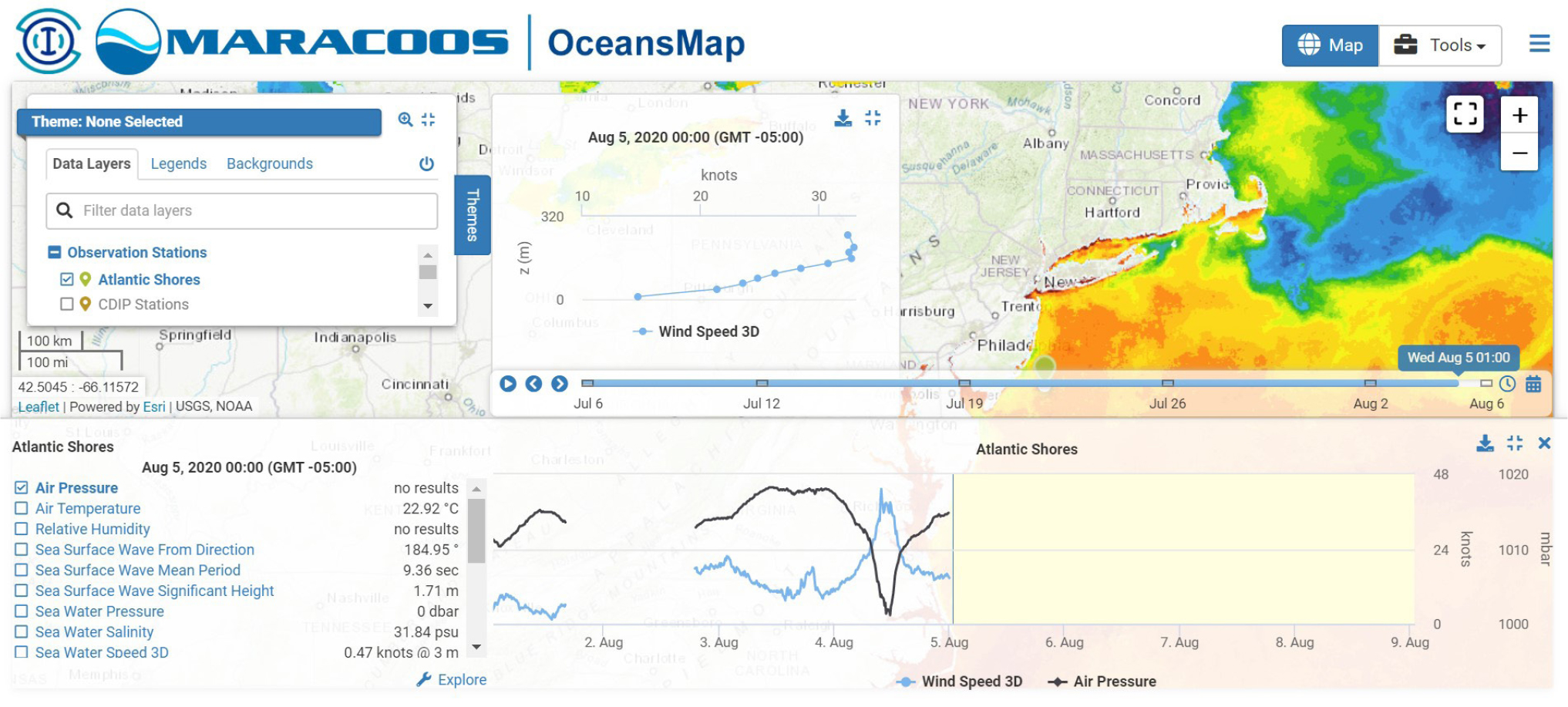
Figure 5. The panel shows a daily data snapshot collected from the Atlantic Shores Offshore Wind LLC buoy deployed off New Jersey as Tropical Storm Isaias passed over on August 5, 2020. Data are available to the public through the MARACOOS OceansMap (https://oceansmap.maracoos.org/). > High res figure
|
Table 2. Existing US data portals that can be leveraged to support management and synthesis of research and monitoring data generated from ongoing and future efforts. > High res table
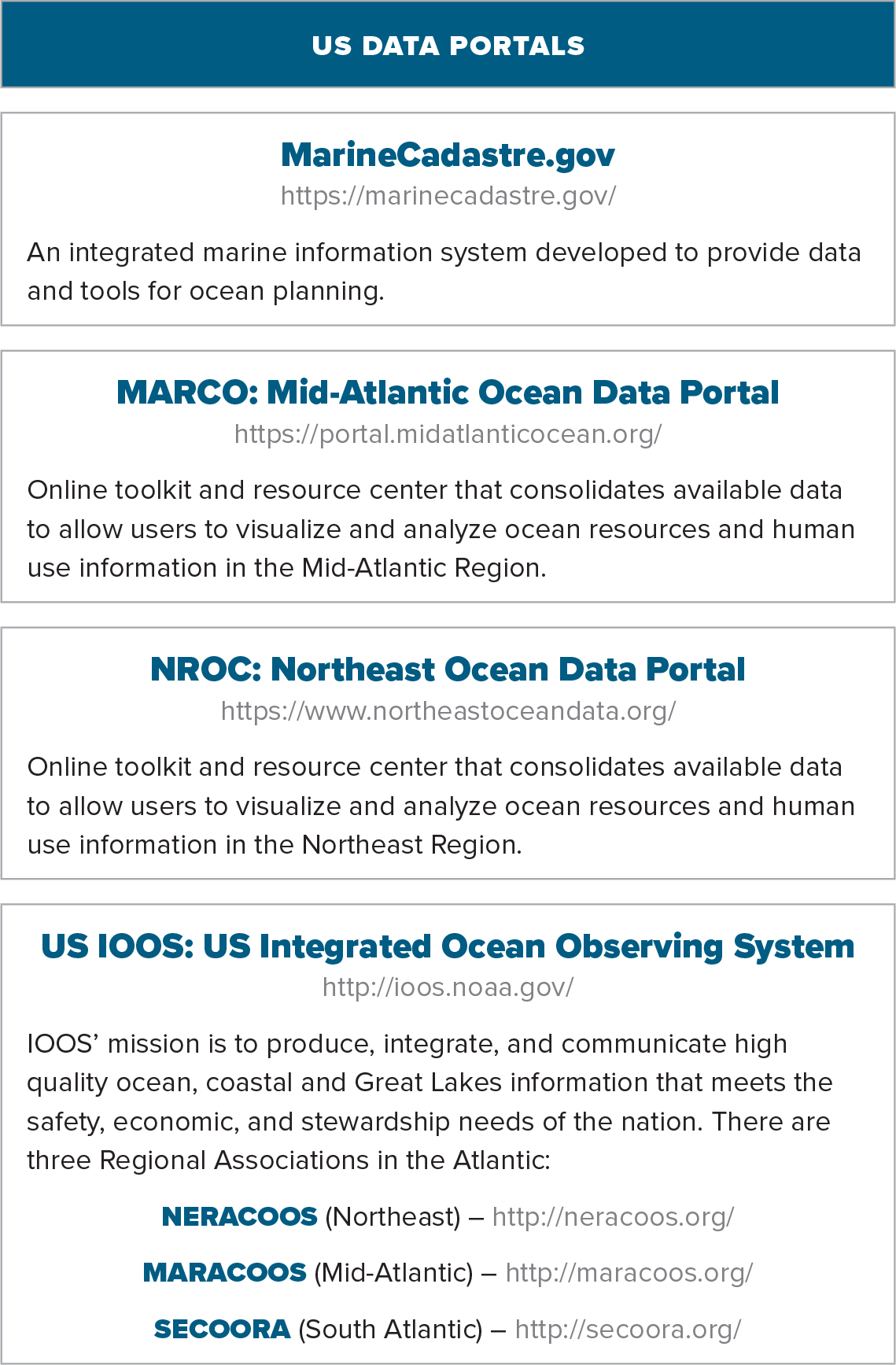
|
DATA AND COLLABORATIVE RESEARCH CONSIDERATIONS
Government agencies, developers, and fishers have recognized the need for improved coordination to advance regional science and research. Fisher concerns and perceptions about impacts to species, habitats, and their activities should be formally documented (e.g., ten Brink and Dalton, 2018) and serve as the basis for hypotheses that can be evaluated with cooperative research programs. Prioritization of directed, hypothesis-driven research designed to address short- and long-term questions is needed, along with transformation of project-specific data into user-friendly information products (RODA, 2020). Cooperative research should be modeled on existing efforts (e.g., Holderness Fishing Industry group in the United Kingdom); co-designed by the agencies, developers, scientists, and the fishing industry; and implemented with fisher involvement throughout all stages.
Experimental design is critical to ensuring that data generated in and around US offshore wind farms can be aggregated at regional levels and synthesized into products that can inform wind developer and regulatory decision-making. Efforts by agency scientists and academic researchers are underway to compare and analyze the benefits and limitations of current monitoring techniques, such as BACI and before-after-gradient (BAG) designs (Methratta 2020). To evaluate changes and their drivers, developers’ monitoring will have to utilize either these techniques or a hybrid approach to study effects over different spatial (e.g., project area versus regional) and temporal (e.g., baseline versus construction monitoring) extents. These issues, and the coordination needed to address them, are being discussed under the concept of a regional monitoring framework—the Responsible Offshore Science Alliance (ROSA, see below).
Holistic ecological research approaches that can address multiple hypotheses should be implemented for 30 or more years of the projects’ “cradle-to-grave” life cycles (leasing, construction, operation, and decommissioning) and used to guide adaptive management. Initial environmental conditions can be documented during pre-installation. Wind infrastructure (turbines) can serve as replicates (controlling for microhabitat) in experimental designs across large-scale environmental gradients, for example, onshore-offshore, water temperature, depth, and habitat type. Hypotheses and predictions regarding specific species’ changes in migration, phenology, distribution and range, recruitment, and connectivity can be developed and tested with long-term monitoring. Turbines can also serve as instrument platforms for monitoring physical parameters (e.g., oxygen), water quality and chemistry, soundscapes (using passive acoustics to detect fish and marine mammals), visual information (with underwater video cameras), migration and site fidelity (using active acoustic telemetry studies), or biomass (employing active hydroacoustics). Resulting data and information can contribute to documenting predicted ecological and ecosystem changes.
Transparent and credible data governance and management are pillars for cooperative research. Common data-sharing platforms can increase the volume of data available for analysis in order to reduce biases and uncertainty that may arise from site-by-site analyses or from the entity collecting the data (e.g., private environmental contractor versus an academic institution). Greater robustness of the data sets in turn will promote engagement and trust among the data generators and the public. Data management structures to support these efforts do not need to be recreated. Instead local, state, and regional efforts can leverage existing federal databases, data management frameworks, and map services and adapt them to support fisheries data (Table 2). Efforts have been initiated among offshore developers, the fishing industry, and governmental agencies to utilize the capabilities of these existing data systems and portals to house, integrate, and synthesize data and information over the lifetime of offshore wind projects.
Responsible Offshore Science Alliance
Formed in 2019, The Responsible Offshore Science Alliance (ROSA) is a first-of-its-kind, cross-sectoral partnership for the New England and Mid-Atlantic regions. It was formed by fishers and wind developers in collaboration with federal and state agencies, academic and nongovernmental institutions, and resource managers (Figure 6). ROSA’s mission is to enhance the scientific understanding necessary to support the coexistence of wind energy development and sustainable fisheries. The organization seeks to accomplish this by convening the scientific community, fishers, wind developers, and government agencies to identify and prioritize research and monitoring needs, coordinate entities, administer research funding, oversee programs, and facilitate dissemination of findings and access to data.
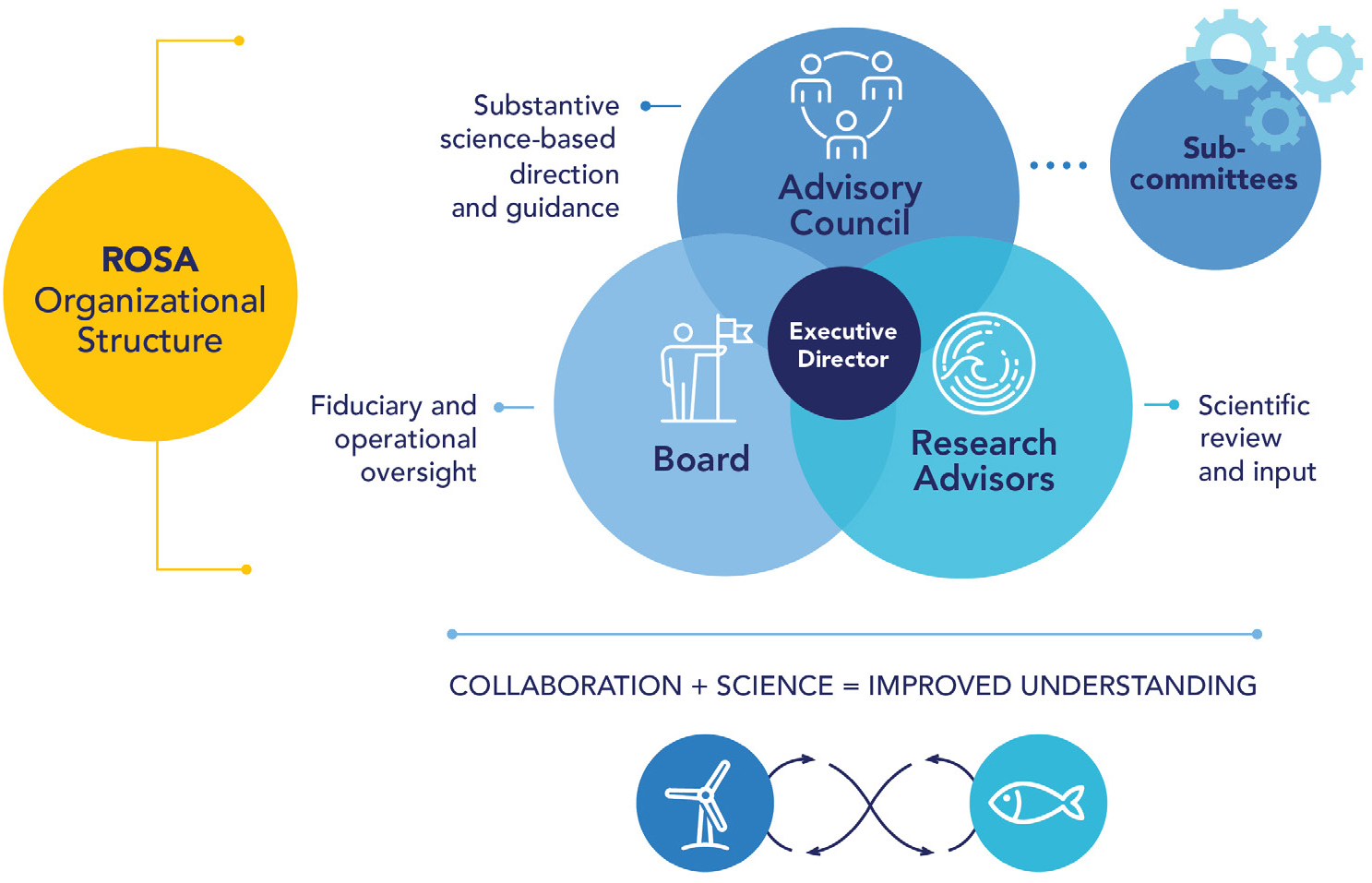
Figure 6. Responsible Offshore Science Alliance (ROSA) organizational structure. Courtesy of ROSA. > High res figure
|
For its first major activity, ROSA developed an interdisciplinary Interim Fisheries Monitoring Working Group to draft monitoring guidance for wind developers. This collaborative approach allows early cross-sectoral participation in the development of guiding principles related to monitoring program objectives, sampling design and methods, analytical tools, and data sharing that will ultimately improve efficiency in the monitoring plan design and review process. The first iteration of guidance was released for public review in October 2020 (ROSA, 2020).
MOVING FORWARD
Offshore wind presents a significant opportunity for economic and governmental entities to address climate change while meeting society’s demand for energy. Scientists and fishers acknowledge that the geographic ranges of marine species can shift, indeed are shifting, in response to climate change in the Mid-Atlantic and New England regions. Changes to fisheries with the presence of offshore wind structures are foreseeable, particularly with regard to reef effects around foundations and scour materials that will be common to US Atlantic offshore wind projects. Evidence from Europe and US wind infrastructure show that there will be reef effects, although these benefits may be not be evenly distributed among all species and fishers in a regional or local area. Reef effects can potentially benefit fisheries and fishers who will work in and around these structures (Figure 7). Identifying fisheries effects from wind infrastructure requires an understanding of these interactions and dynamics, recognition of the need for coexistence of offshore wind and fisheries, and concerted and collaborative efforts and data sharing across all groups to establish necessary hypothesis-driven research priorities and standardized monitoring techniques.
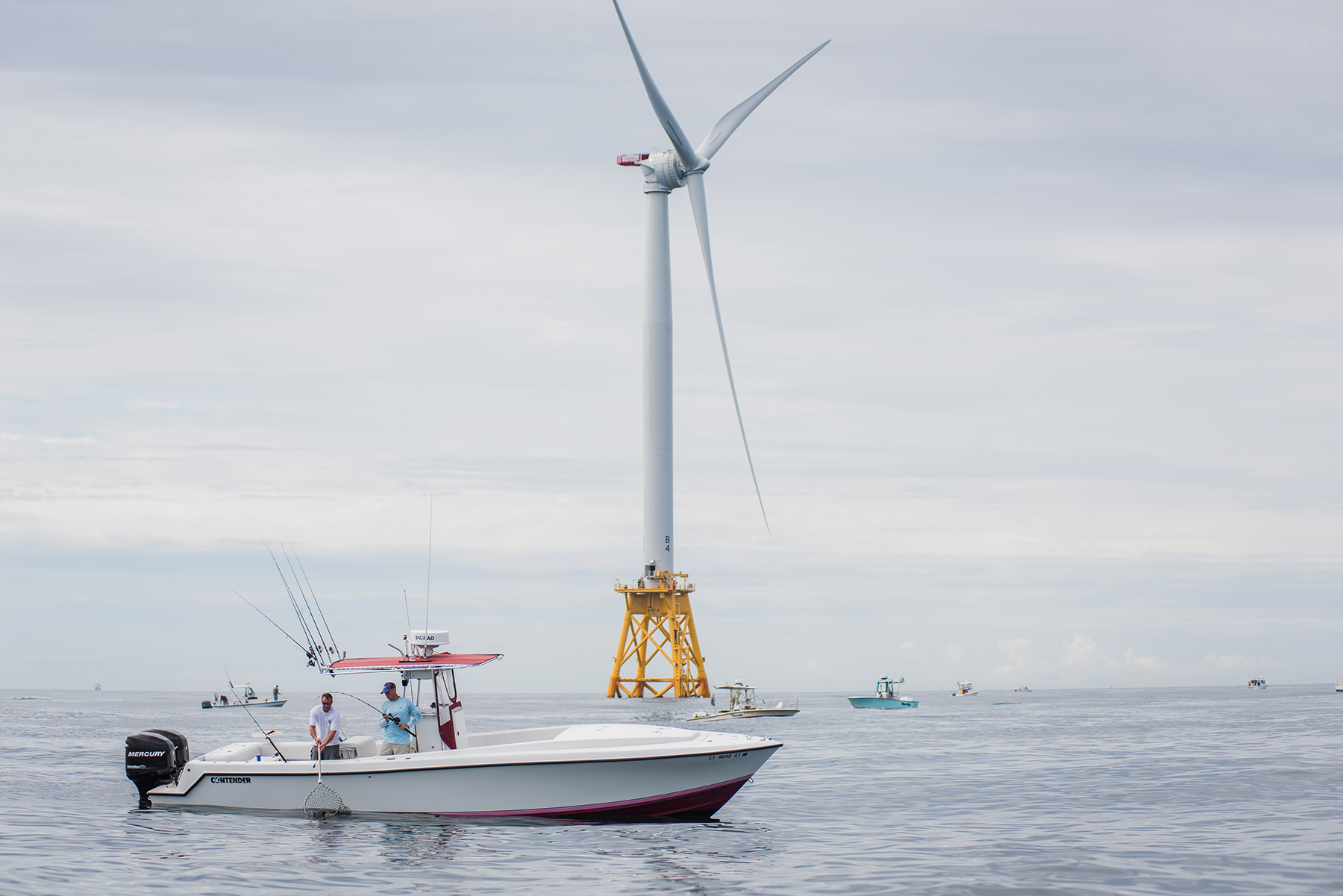
Figure 7. Fishing activity in and around the Block Island Wind Farm off of Rhode Island, USA. Courtesy of Ørsted. > High res figure
|
Recent efforts among government agencies and the offshore wind and fishing industries are promising to revolutionize the process of generating, aggregating, and sharing data collected during offshore wind development phases and to assess how impacts can be locally and regionally mitigated. ROSA is pioneering partnerships among entities, prioritizing research, standardizing monitoring, and sharing data and synthesis at local, state, and regional levels to enhance the relationship between offshore wind and fisheries. The life spans of offshore wind infrastructure and possibilities for instrumenting the structures provide opportunities to support ecosystem-based research approaches and adaptive management. Furthermore, these combined efforts will create the ability to assess changes in marine ecosystems and associated fisheries in relation to an emerging industry, a changing climate, and other factors over the next 50 years.
These opportunities and pathways exist; fully utilizing them requires trust and transparency between the offshore wind and fishing industries. Government agencies also have a lead role in helping to build this trust. Success, in fact, relies on the trust built among all parties. Combined, these efforts and the trust built will create an unprecedented US leadership opportunity for demonstrating how two critically important ocean resources—ocean renewable energy and fisheries—can be responsibly managed to allow functional coexistence of communities dependent on both.
ACKNOWLEDGMENTS
This synthesis addresses many topics, and the authors would like to acknowledge the contributing organizations: Vineyard Wind, Ørsted, Mayflower Wind, New England Aquarium Anderson Cabot Center for Ocean Life, ROSA, Responsible Offshore Development Alliance, BOEM, NOAA (Northeast Fisheries Science Center and IOOS), SeaJay Environmental, Massachusetts Lobstermen’s Association, and the University of Massachusetts Dartmouth School for Marine Science & Technology.