Synchrotron Instruments
Synchrotron light sources are large, circular particle accelerators that run continuously to generate very bright light. Beamlines are built tangent to the storage ring to receive this synchrotron radiation. Mirrors and monochromators along the beamline focus the light and tune its wavelength, so that experiments can be done with a small spot and well-defined energy that permits fluorescence and absorption measurements. Samples and detectors are mounted at the end of beamline in the endstation, where experiments are set up and measurements are collected. Synchrotron facilities in use today vary from tens to hundreds of meters in diameter. Most synchrotron radiation that is harnessed for science is light in the X-ray part of the spectrum, but there are also ultraviolet and infrared synchrotron instruments.
The accelerator, storage ring, and beamlines are all heavily shielded to protect people, equipment, and the environment from the synchrotron ionizing radiation. A system of interlocks is in place to allow people to shut off the X-rays and mount their samples in the endstations. Experiments are controlled from outside of the endstation. There is no way to enter the endstation while the X-ray shutters are open, and there are several ways to quickly stop the X-rays.
A description of currently operating synchrotrons around the world and their instruments can be found at https://lightsources.org/. Synchrotron facilities typically operate 20–40 beamlines simultaneously and independently. Beamlines have varied capabilities and a defined range of energies that define what elements they can detect. Synchrotron instruments are grouped by their wavelength or energy range: (1) “soft” X-ray beamlines measure elements such as C, N, and O (~200–1,000 eV); (2) “tender” X-rays beamlines measure elements such as S, P, Al, and Si (~1,000–4,000 eV); and (3) “hard” X-ray beamlines measure Ca and up through the transition and heavy metals (~4,000+ eV). Multiple spatial scales are available within these energy groupings, from bulk instruments that have a beam footprint of several millimeters to micro- and nanoprobe instruments that have micrometer-range spot sizes.
Synchrotron Uses in Marine Science
Synchrotron instruments are relevant for marine science because they measure chemical composition and speciation of elements at low concentrations (tens of parts per million) in small sample quantities (<<<1 g). Methods are generally nondestructive, so several kinds of measurements can be made on the same sample at different beamlines in addition to using other lab-based techniques. Synchrotron measurements are broadly grouped into those used to study the absorption, transmission, or scattering of light when it interacts with matter. Non-synchrotron analogs of these instruments exist, such as benchtop X-ray diffraction (XRD) instruments and light and electron microscopes. Synchrotron methods offer greater sensitivity and quantitative measurements, which can be difficult to obtain with X-ray fluorescence (XRF) generated by SEM-EDX (scanning electron microscopy with energy dispersive X-ray spectroscopy). Importantly, synchrotron XRF is more sensitive for trace elements than SEM-EDX, and the incident energy of the X-rays can be controlled, which allows for element-specific chemical speciation measurements.
Common synchrotron techniques for marine science are XRF, X-ray absorption spectroscopy (XAS), scanning transmission X-ray microscopy (STXM), and Bragg diffraction (XRD) experiments (Toner et al., 2016a). XRF is used to analyze spatial relationships and quantitatively map co-occurrence of elements (Toner et al., 2016b; Twining et al., 2021). XAS detects the valence state and bonding environment of elements of interest (Sutton and Newville, 2013; von der Heyden, 2020). STXM can measure both light elements and the L-edge of transition metals (Figure 1; Hoffman, 2018; Hoffman et al., 2018; Brandes, et al., 2010). XRD detects repeating patterns of atoms in well-crystalline materials, creating a diffractogram output that is compared to a database to identify the compounds that make up a sample under the X-ray beam (Figure 2; Dinnebier and Billinge, 2008; Dunlap, 2018; Callefo et al., 2019; von der Heyden, 2020).
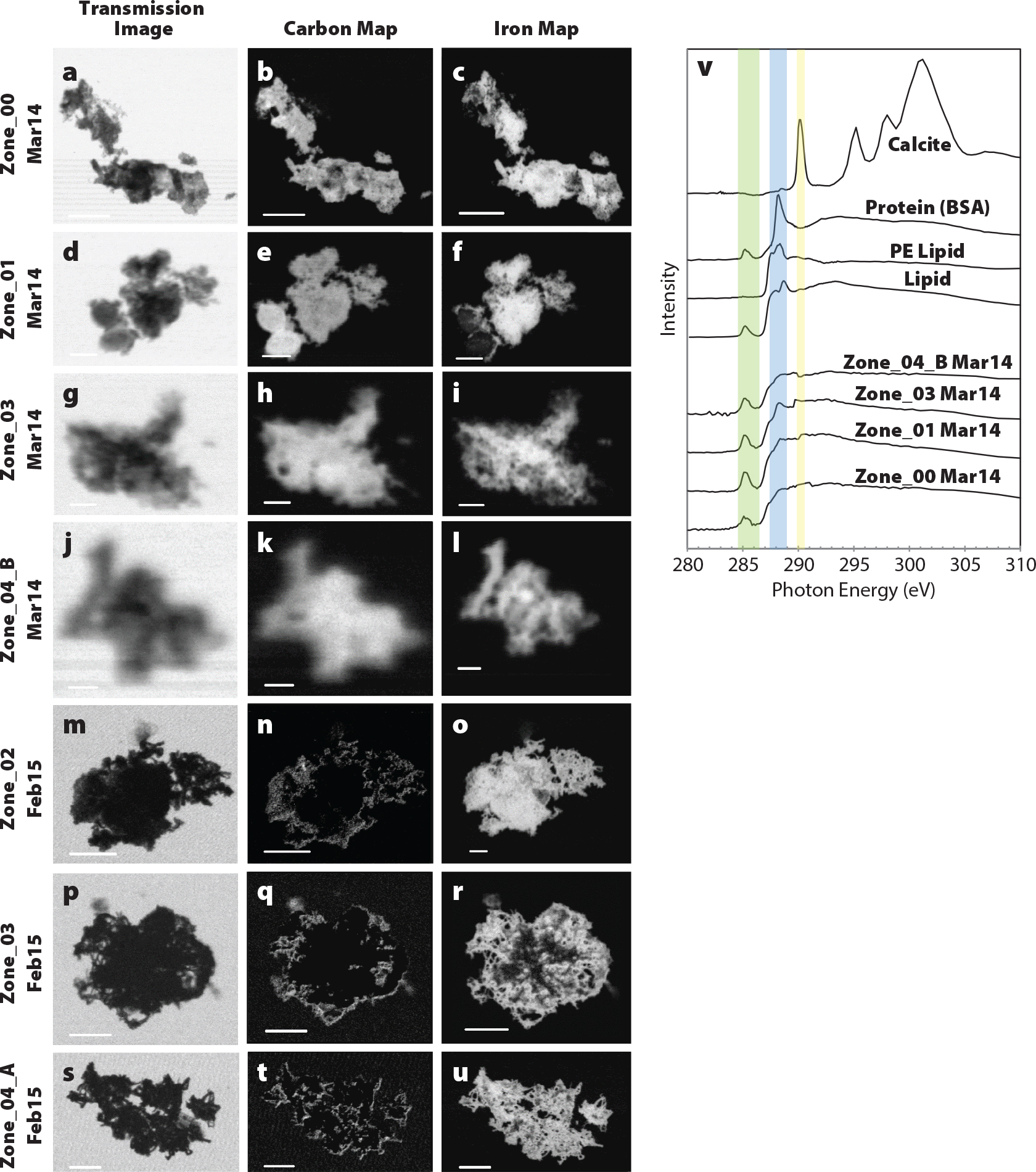
FIGURE 1. All marine particles analyzed in the middle of a hydrothermal plume at Station 20 (2,550 m depth) from the GEOTRACES-East Pacific Zonal Transect (GP16). Transmission images were collected at 290 eV (a, d, g, j, m, p, and s). Scale bars for (a–c, m–u) are 5 µm, (d–f) are 2 µm, (g–j) are 1 µm, and (j–l) are 500 nm. Carbon 1s XAS spectra from the middle of the plume at station 20 were compared to standards (Brandes et al., 2010; Chan et al., 2011). (v) The green bar corresponds to the ~285 eV peak, the blue bar highlights the region for the carbonyl peak, and the yellow bar highlights the 290 eV peak for inorganic carbon. Zone_01 Mar14 was previously published in Fitzsimmons et al. (2017); this figure was adapted from a supplemental figure in Hoffman et al. (2018). Images and spectra collected at the Advanced Light Source Polymer Scanning Transmission X-Ray Microscopy (STXM) beamline. > High res figure
|
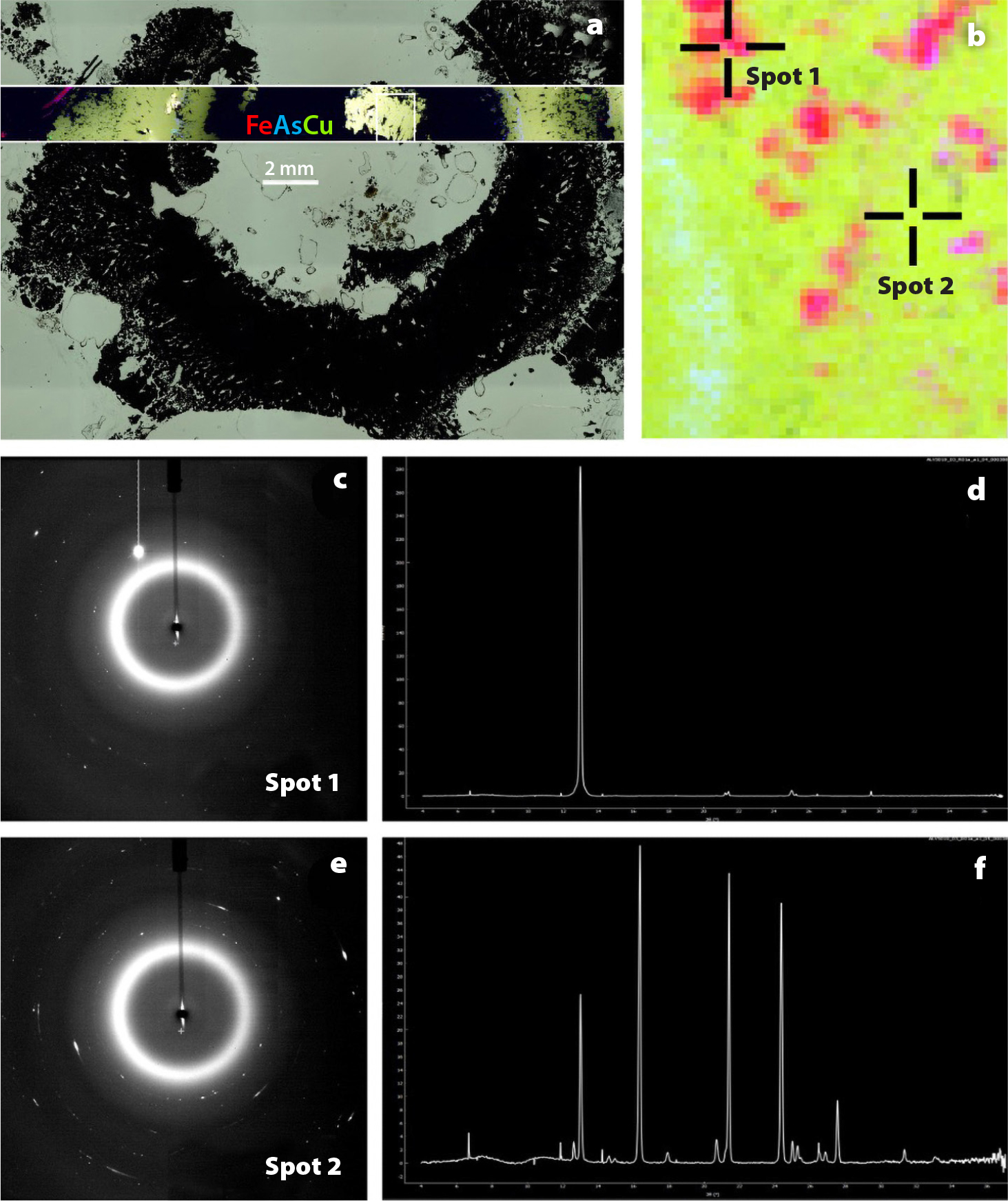
FIGURE 2. (a) X-ray fluorescence (XRF) map of hydrothermal vent mineral sample element distribution imposed on light-microscope image of the same sample (East Pacific Rise; collected on cruise AT42-09 2019) showing iron in red, arsenic in blue, and copper in green. (b) Closeup XRF image of the detail area marked in the box in panel (a), with iron in red, arsenic in blue, and copper in green. (c) X-ray diffraction (XRD) diffractogram, and (d) radially integrated pattern of spot 1 on image in panel (b). Mineral identified as chalcopyrite by Match! software. (e) XRD diffractogram, and (f) radially integrated pattern of spot 2 on the image in panel (b), mineral identified as kesterite by Match! software. Data from Jones and Toner (unpublished). Diffractograms and spectra generated by Dioptas software. XRF and XRD collected at the National Synchrotron Light Source II XFM beamline. > High res figure
|
How to Access Beamline Instruments
To access a synchrotron beamline, scientists write a short proposal describing their hypotheses and their planned experiments. Beamline scientists can provide guidance to researchers (“users”) about experimental design, appropriate instruments, and planned measurements before the proposal is submitted. Proposals are then peer reviewed and evaluated for feasibility. Successful proposals are allocated beamtime, typically two to four days repeated two to three times a year. During beamtime, beamline scientists help users collect and analyze their data. Synchrotron beamtime for science is free for users, and in some countries travel expenses may be covered. Light sources typically have two to three proposal cycles per year, with different proposal deadlines. Most light sources offer introductory workshops that cover specific types of experiments.
Remote beamtime has increased since the global Covid-19 pandemic. This varies from mail-in experiments, where samples are sent to the beamline and fixed sets of measurements are collected, to fully remote experiments, where samples are introduced to the beamline endstation by beamline scientists and the instruments are controlled remotely by the users. Most remote experiments fall somewhere in between, with measurements collected by the beamline staff with guidance from the users.
Guidance for Successful Synchrotron Analysis
1. Selecting the right technique is important for complex and heterogeneous marine science samples.
Marine samples are complex and heterogeneous, and no single element will reveal everything about a process of interest. Experts are available to help with the critical considerations of selecting beamline instruments appropriate for the sample, the spatial scale required, and the concentration of elements under consideration, and they should be consulted before samples are collected. For example, one consideration is whether a study will use bulk or spatially resolved probe instruments, as each method requires different experimental approaches and provides different data outcomes.
Bulk measurements have millimeter-scale spatial resolution. They are generally higher throughput than focused-beam techniques because the area illuminated by the beam is larger (Lam et al., 2015; Shoenfelt et al., 2018a). The most detailed speciation results require samples where the crystalline constituents are small and in a random orientation (Dinnebier and Billinge, 2008a; Dunlap, 2018). Several grinding and other preparation steps, each of which increases the chance of contamination, may be needed to obtain the correct crystal samples. Bulk measurements can also be used on heterogeneous samples to determine average speciation or redox states of the area illuminated. This can be a good strategy for determining large-scale changes in the environment (Lam and Bishop, 2008; Lee et al., 2021; Xiang et al., 2021).
Micro- and nanoprobe instruments use focusing mirrors and lenses to create small beams for imaging of heterogeneous samples and mapping spatially resolved chemical speciation measurements on micro- or nanoscale features within the sample. They can discover spatial heterogeneity in a sample at different scales, but each sample takes longer to measure when compared with a bulk experiment.
Other challenges to obtaining good measurements include instrument detection limits, beam damage, and sample heterogeneity at small spatial scales. Consulting beamline scientists early in the experiment design process will help users choose the right instruments, and they can identify and mitigate potential challenges.
2. Appropriate collection and preservation methods are needed for sample preparation method and synchrotron technique.
Samples must be collected and preserved appropriately to ensure good quality data. The heterogeneity, complexity, and range of sample types of natural materials mean that although sample preparation is important, the best approach varies between studies (Steele et al., 2019). Each additional preparation, preservation, and transport step can lead to contamination (Cibin et al., 2019) and other artifacts.
Our workshop speakers highlighted contrasting approaches. Twining et al. (2021) captured and preserved individual cells by centrifuging them onto the measurement substrate at sea to avoid contamination by dust (Figure 3), and great care was required to remove salt and to stabilize the sample for measurements while reducing loss of elements (as from excessive rinsing with deionized, or DI, water). Hoffman et al. (2018) preserved iron speciation of their marine particles by carefully minimizing sample exposure to oxygen at each step, from collection at sea to measurement at the beamline. In contrast, Shoenfelt et al. (2018) presented high-throughput bulk Fe XAS data with no specific preservation of samples so they could process hundreds of XAS data points from archived samples to cover long timescales. This approach was successful due to the low likelihood that the target Fe species (iron silicates and oxides) would change in dry storage. Again, consulting beamline scientists early in the experiment design process can help with choosing the right approach.
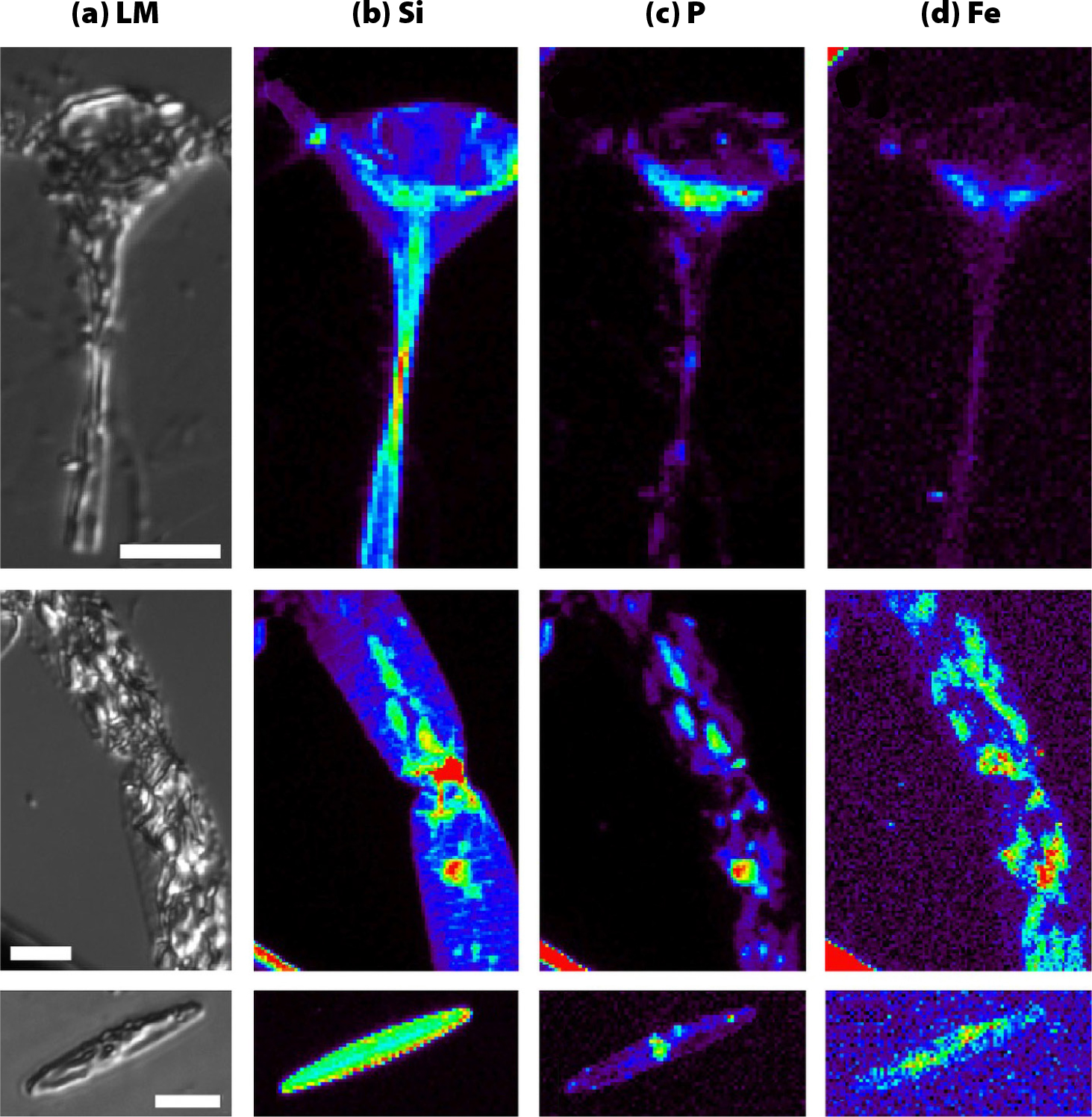
FIGURE 3. Images of phytoplankton cells (a) light microscope images. (b–d) XRF element maps showing the distribution of (b) silica, (c) phosphorous, and (d) iron. Data from author Twining (unpublished). > High res figure
|
3. Different techniques require different sample preparation methods.
Options and pitfalls of sample preparation for the selected instrument and technique depend on sample collection and preservation methods, and the desired outcome of a study. However, background interference is a common pitfall for all studies. Care in the removal or minimization of elements of interest in the mounting materials and the use of external and internal standards greatly increases the quality of data that can be collected.
Soft and tender X-ray mounting techniques require care due to the ubiquity of C, Cl, and S in many adhesives and epoxy resins. The main sample mounting preparations for soft X-rays are silicon nitride membrane and transmission electron microscopy (TEM) grids. For silicon nitride membranes, samples are deposited by drop casting. However, formation of salt crystals as water evaporates from the samples can break the fragile membranes and obscure target sample features. TEM grids support the sample across openings or voids where observations are made in transmission mode. TEM grids are usually less expensive and less fragile than silicon nitride membranes. Samples on silicon nitride membranes and grids can be measured in fluorescence or transmission modes, making them suitable for measurements with multiple instruments (i.e., “multi-modal”). Tender measurements can be made on thin or thick samples because the effective sampling depth is only a few millimeters (Northrup, 2019).
Hard X-ray bulk and microprobe sample preparation is generally simpler than for soft X-rays because samples collected on filters can be loaded directly onto the sample holder (Hoffman et al., 2018). However, filter composition can be a source of background interference (Lam et al., 2006), and a heavily loaded filter may be thicker than is optimal and produce distorted data. Thin sections are an alternative to filters (Marcus et al., 2015). This method can also be used for delicate samples by embedding the sample in low-sulfate resin epoxy before thin-sectioning onto quartz petrographic slides to preserve sample orientation and reduce background interference. Preparation of thin sections is more involved than preparation for filters, but thin sections can allow better and easier measurements. This preparation method can be used for multiple non-destructive microscopy and microprobe techniques. There are many commercial thin section services.
The details of sample preparation, including loading of material on filters and total thickness of samples, impact the quality of measured data (Calvin, 2013). X-ray penetration depth through the sample and substrate is particularly relevant for transmission measurements, but it is also an important consideration for fluorescence measurements, as thick samples may self-absorb fluorescence X-rays. The effects of sample thickness can be calculated using look-up tables for X-ray absorption cross sections (Elam et al., 2002), and samples can be optimized accordingly. Beamline scientists can provide guidance for users on sample preparation.
Example Case Studies
We include case studies, drawn from our workshop, as examples of how various techniques and approaches can be used with a variety of samples to answer marine science questions. Several beamline scientists attended and participated in discussions, highlighting themselves as willing resources and collaborators throughout the process. Links to recordings of the original workshop talks are provided in the online supplementary material.
Brandy Toner (University of Minnesota) provided advice on combining measurements from multiple instruments, approaches, and elements to understand large-scale marine and global processes across time and space, using examples from her work on the speciation of marine hydrothermal iron (Toner et al., 2016a; Stewart et al., 2021).
Alessandra Leri (Marymount Manhattan College) studies the interactions of chlorine, bromine, calcium, and iron in marine particles (Leri et al., 2010, 2015). Her work presents an example of the importance of reducing the background signal to get contrast in the element of interest. She described an exhaustive sample washing process to reducing background levels of inorganic halides from seawater without altering organic matter composition. Her work requires avoiding Cl and Br found in most mounting materials, and multi-energy mapping to distinguish between aliphatic and aromatic organochlorine (Leri et al., 2010, 2015).
Colleen Hoffman (University of Washington) used multi-element STXM and associated XAS to decipher the organic-mineral relationship of marine particles (Hoffman et al., 2018; Hoffman, 2018). Her work used the multi-element capability of STXM techniques to provide co-located measurements of light elements like carbon and nitrogen with transition metals like iron, copper, and sulfur.
Wen Hu (NSLS-II) presented an overview of a new soft X-ray microscope beamline called Soft X-ray Nanoprobe (SXN) that is being built at NSLS-II. SXN will have a fluorescence detector and a total electron yield (TEY) detector, as well as transmission detectors, making it possible to monitor multiple elements from a sample via fluorescence while collecting element-specific transmission and chemical speciation maps on a nanometer scale.
Phoebe Lam (University of California Santa Cruz) uses hard X-ray bulk and microprobe methods for understanding cycling in the ocean of Fe and Mn, which are both essential micronutrients as well as great scavengers of other trace elements and isotopes (Lam et al., 2006). Her work provides an example of overcoming challenges associated with the heterogeneous nature of marine particles collected by in situ filtration.
Ben Twining (Bigelow Laboratory for Ocean Sciences) measures levels of transition metals within individual marine phytoplankton cells for understanding how they obtain macronutrients (Twining et al., 2021; Figure 3). As phytoplankton metal contents are affected by many environmental factors and are difficult to extrapolate from laboratory cultures, Twining described his methods for preparing phytoplankton cells collected directly from the ocean. This work also demonstrates how to quantify synchrotron elemental analysis using measurement error and precision, along with external and internal standards.
In contrast, the work of Ben Bostick (Lamont-Doherty Earth Observatory) is an example of using high-throughput bulk XAS data from legacy marine cores (Shoenfelt et al., 2018) to show the effects of glacial dust on global productivity over the last 140,000 years. Resolving mineralogical differences on this scale requires a statistically relevant volume of samples and consideration of how different chemical species change during long-term storage of the original cores.
Summary
Experienced users within the marine science community presented advice on and examples of how to use synchrotron measurements to answer research questions. Major considerations discussed for conducting successful studies included instrument capabilities in relation to elements of interest and spatial scales, concentration of elements of interest, and planned techniques. Beamline scientists are valuable resources for advice and help in planning experiments to optimize sample collection and preservation for synchrotron analysis and should be consulted as early as possible in experimental design. Getting the best results from synchrotron measurements requires incorporating sample preservation, preparation, and instrument capabilities into experimental design before getting on a ship to collect samples.
Acknowledgments
This conference was organized by the Users’ Executive Committees of the National Synchrotron Light Source II (NSLS-II) and the Center for Functional Nanomaterials (CFN) on behalf of NSLS-II and CFN, two US Department of Energy (DOE) Offices of Science User Facilities operated for the DOE Office of Science by Brookhaven National Laboratory under Contract No. DE-SC0012704. Further support was provided by sponsors listed at https://www.bnl.gov/nslscfnum/events/2021/sponsors.php.
Author Contributions and Potential Conflicts of Interest
RJ, SN, and PN organized the workshop. BB, CH, WH, PL, AL, BMT, and BST presented their data and insights at the workshop. The manuscript was written by SN and RJ with corrections and edits from the other authors. RJ, CH, and BST contributed figures for the manuscript. None of the authors have any financial interests that could be perceived as being a conflict of interest.