Introduction
I was raised among the rolling landscape of the Texas Hill Country in the United States. Above my home, the powerful squalls arising from springtime heat over the Gulf of Mexico brought great rains that spawned wildflowers and greenery among the ancient deposits of granite and limestone. As these fresh waters filled the Colorado River on its journey seaward, they accumulated ions, tannins, and nutrients. My understanding of this process expanded when I enrolled in Introductory Oceanography at Texas A&M University and I learned that rivers, like the Colorado, are one critical source of micronutrient trace metals (Mn, Fe, Co, Cu, Zn, and Cd) to the ocean. Near the end of that semester, I heard about the GEOTRACES program for the first time when Laramie Jensen gave a guest lecture describing the US GEOTRACES GN01 cruise to the North Pole (Jensen et al., 2020; Jensen and Colombo, 2024, in this issue). GEOTRACES is an international effort to elucidate the biogeochemical cycles of trace elements and their isotopes (TEIs) in every ocean basin by utilizing chemical proxies to understand both modern and paleo circulation, productivity, and climate (Anderson et al., 2014). Trace elements are essential to marine life, and their rapid cycling in the euphotic zone controls phytoplankton community assemblages, thus ultimately governing marine ecosystems and global primary production (Morel and Price, 2003). During Jensen’s lecture, I discovered that GEOTRACES scientists seek to improve our understanding of TEI source and sink fluxes, internal cycling, chemical speciation, and biogeochemical transformations along ocean-ocean and ocean-atmosphere interfaces using process-relevant tracers (e.g., Fe and Mn for redox processes). And, once these tracer data are constrained, they use them to map TEI distributions (Schlitzer et al., 2018), identify local- and basin-scale trends in TEI cycling, and quantify source fluxes, internal cycling, residence times, and paleoproductivity estimates (Hayes et al., 2015; Anderson, 2020; Horner et al., 2021), which contribute to inverse and Earth system model forecasting of trends in ocean and climate health (John et al., 2020; Tagliabue et al., 2020).
As the fall semester turned to spring and summer, I delved deeper into GEOTRACES and pursued two undergraduate research projects. Working with Jessica Fitzsimmons at Texas A&M, I helped analyze samples from the US GEOTRACES GP15 section to identify sources and cycling of dissolved Fe, Mn, Cd, and Pb in the upper 1,000 m of the North Pacific. And as a Research Experiences for Undergraduates (REU) student with Ben Twining at Bigelow Laboratory, I used US GEOTRACES GA03 and GP16 section data to compare decoupled micronutrient ratios in the Atlantic and Pacific basins. These experiences exposed me to the rewards of life spent in research. So, I accepted a PhD position at the University of South Florida working with Tim Conway, taking on two GEOTRACES projects that employed metal stable isotope ratios (δ56Fe, δ60Ni, δ66Zn, and δ114Cd) to tease apart the individual processes that set the distributions of dissolved Ni in the Arctic-North Atlantic (GAPr16) and dissolved Fe, Zn, and Cd in the Pacific sector of the Southern Ocean (GP17-OCE).
International Science
I have been fortunate that my graduate career has since taken me to GEOTRACES events around the world. In July 2022, along with 47 other students representing 16 countries, I participated in the third international GEOTRACES Summer School at the Alfred Wegener Institute (AWI) in Bremerhaven, Germany. This five-day workshop included interactive lectures, laboratory exercises, student networking opportunities, and lessons in the framework of GEOTRACES data production, processing, intercalibration, and management, with emphasis on cultivating GEOTRACES’ underpinning values: inclusivity, diligence, and collaboration. This experience was rich in cultural exchange, making it personally and professionally transformative. AWI’s classroom provided an inclusive space for international perspective and inquiry, where students were given time to discuss biogeochemical hypotheses with like-minded, driven peers during poster sessions, seagoing practicals, and social hours. Events such as the GEOTRACES summer school are one of the program’s many strengths. These events provide the next generation of scientists with the opportunity to learn program fundamentals from leaders in the field, deepen their biogeochemical competencies, and build international relationships, thereby invaluably strengthening the corps of investigators in the GEOTRACES network (Frank et al., 2003) and beyond.
In November 2022, I began my position as a GEOTRACES carousel (GTC) supertech on the National Science Foundation (NSF) US GEOTRACES GP17-OCE cruise. I stepped onto the Nimitz Marine Facility pier in San Diego, California, to the sound of hydraulics lifting the shipping containers and scientific equipment that would become home, family, and adventure. Every hand was in motion preparing R/V Roger Revelle for a 57-day cruise from Papeete, Tahiti, to Punta Arenas, Chile. There was excitement in the air, for GP17-OCE was planned to significantly supplement the scarce dissolved (≤0.2 μm) and particulate (≥0.2 μm) Mn, Fe, Ni, Cu, Zn, and Cd data, including δ56Fe, δ60Ni, δ65Cu, δ66Zn, and δ114Cd, previously collected in this vast section of the South Pacific and Southern Ocean. This transect included high-resolution sampling throughout the hyper-oligotrophic waters of the South Pacific gyre (Claustre et al., 2008) and the fronts of the Antarctic Circumpolar Current (Orsi et al., 1995). GP17-OCE was planned to span ecologically and hydrographically important productivity gradients, deep-water formation regions, and the previously unexplored Pacific-Antarctic Ridge. When synthesized, these water column, aerosol, and porewater data will help describe the control that TEI cycling has on South Pacific and Southern Ocean phytoplankton ecology, micronutrient speciation, and global carbon cycling.
Following the welcome brief by Chief Scientists Ben Twining, Jessica Fitzsimmons, and Greg Cutter, we departed from cyan Tahitian waters in December, sailing out of the tropics and into the core of the South Pacific subtropical gyre. Revelle steamed onward toward the Antarctic Convergence Zone amid the gales and storms of the Roaring Forties, Furious Fifties, and Screaming Sixties. Through it all, the bridge held stations steadfastly, allowing us to prepare, winch, and recover the trace metal rosette from the deepest reaches of abyssalpelagic waters (Figure 1). Meanwhile, the GTC team worked diligently at each station, readying hundreds of acid-cleaned bottles for hours of seawater sampling (Cutter and Bruland, 2012). The GEOTRACES van became a meticulously clean world of its own. Here, five of us donned polyethylene gloves and rubber clogs to sit shoulder-to-shoulder for hours on buckets, repetitiously drawing dissolved and particulate TEI samples from the CTD rosette’s 23 Teflon-coated, 12-liter General Oceanic GO-FLO bottles affixed to the walls. Sampling sessions were filled with enlightening stories and laughter. The rest of the science party welcomed this camaraderie at meals, where an open seat meant an opportunity to trade sea stories and hypotheses.
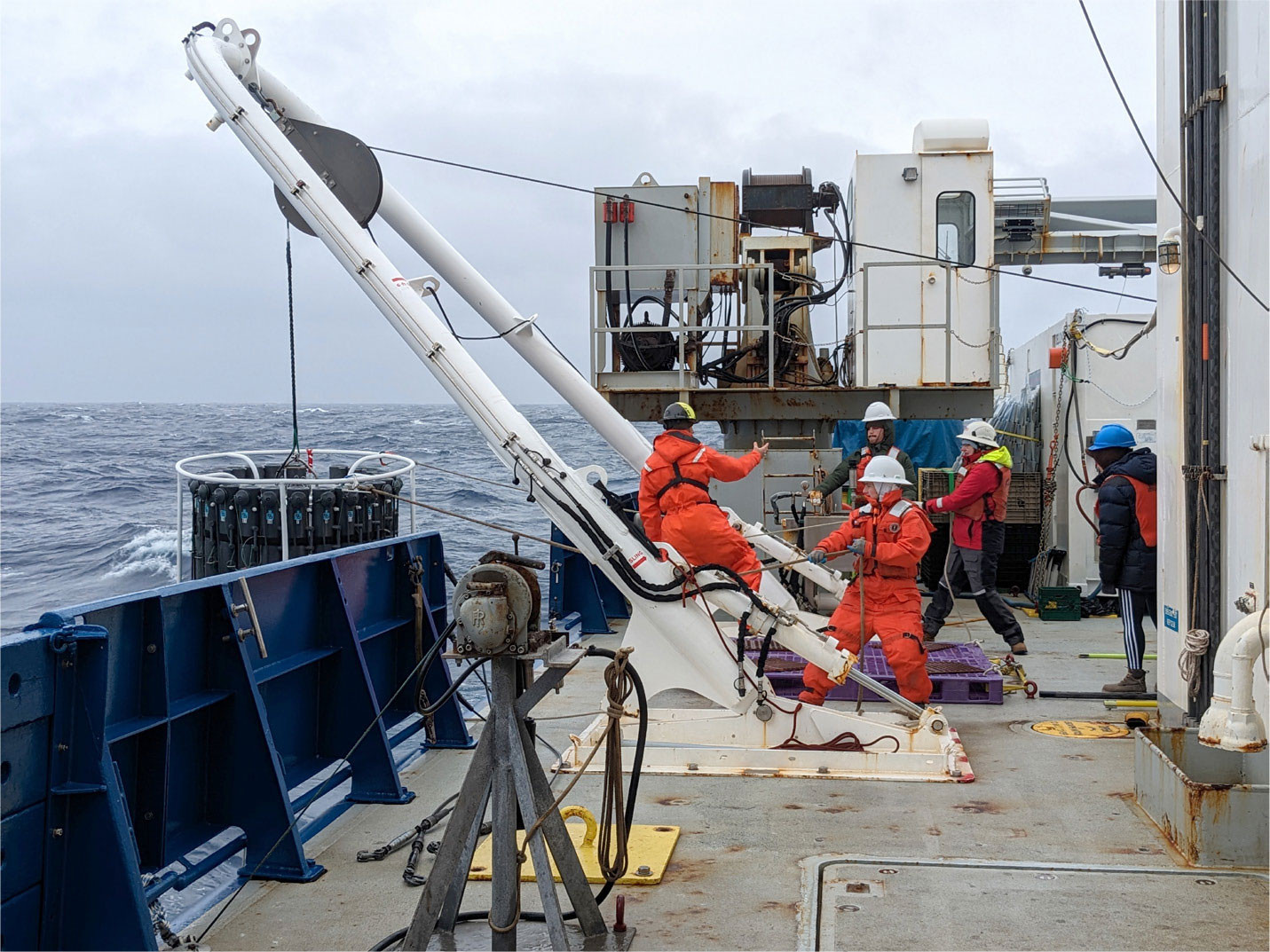
FIGURE 1. From left to right, Mason Schettig (Resident Technician) and GEOTRACES carousel (GTC) team members Laura Sofen, Dylan Halbeisen, Emily Frett, and Yerim Kim recover the GTC trace metal rosette (team members Phil Kong and Jessica Fitzsimmons [Co-Chief Scientist] are off-camera coordinating winch operations). Photo credit: Jennifer Middleton, Lamont-Doherty Earth Observatory. > High res figure
|
Setting course southeastward, Revelle crossed the Antarctic Circle, occupying stations under the midnight sun. Increasingly, towering icebergs appeared on the horizon, but regardless of the cryospheric conditions, work persisted (Figure 2). Conductivity, temperature, depth (CTD) sensors, GO-FLO macronutrient analysis, and flow cytometry corroborated signals of Circumpolar Deep Waters, upwelled nutrients, and diatoms. Once filtered, requested seawater samples were either carefully packaged for post-cruise transport to onshore laboratories or delivered to onboard scientists measuring their specific parameters. Shipboard flow-injection analysis, cathodic stripping voltammetry, Ra delayed coincidence counting, Th anti-coincidence delayed beta counting, and imaging flow cytometry, coupled with salinometry and macronutrient analyses produced preliminary dFe, dMn, dAl, dZn, 224Ra, 234Th, hydrographic, and phytoplankton taxa data each week. These data depicted novel distributions, which helped guide sampling and stimulated discussions among scientists on the vessel. I found that these conversations kept morale high during periods of arduous work, validating the ever-present GEOTRACES culture taught in Germany earlier that year.
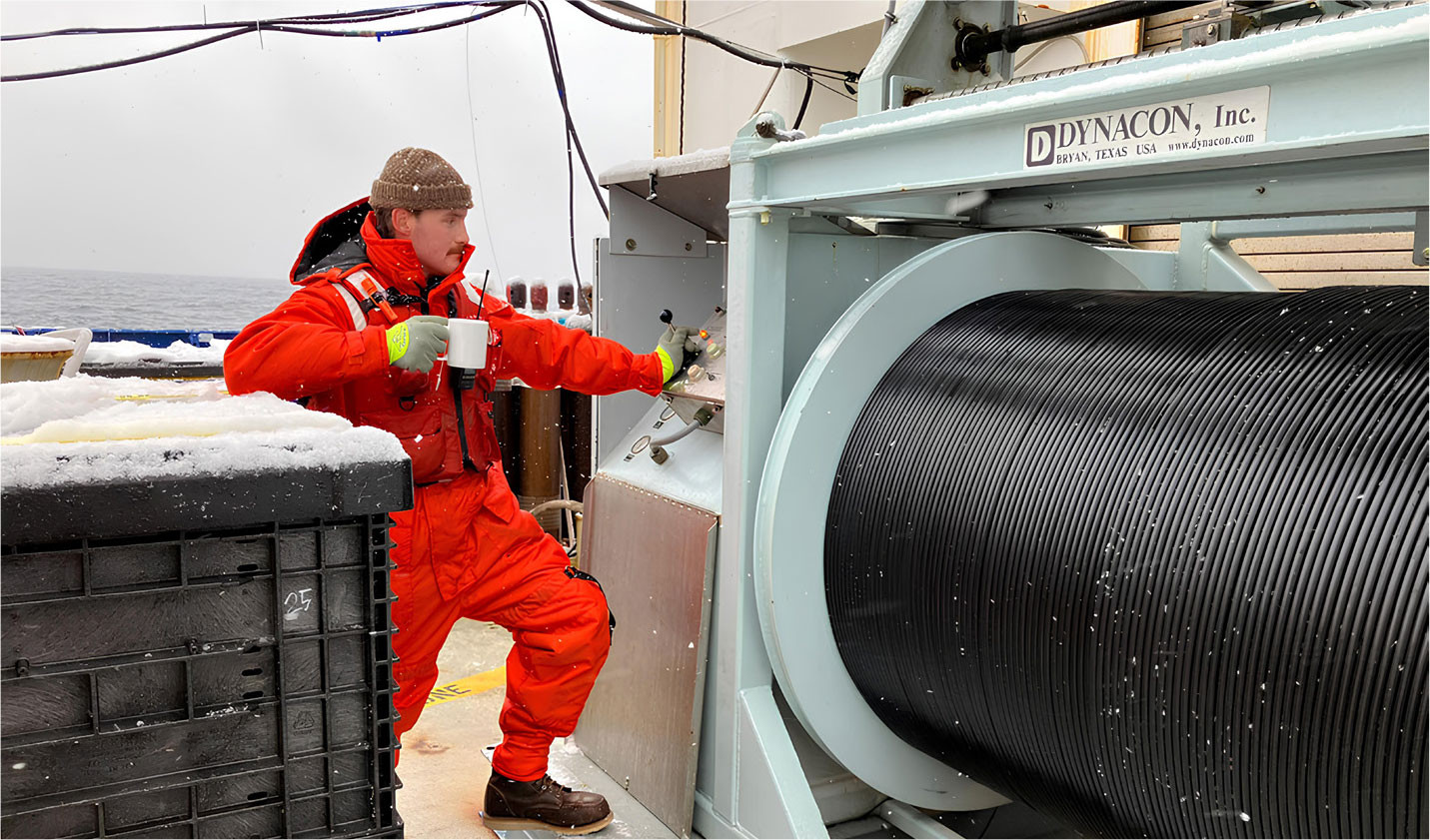
FIGURE 2. Along 67°S, author Dylan Halbeisen descends the GTC rosette to depth during a snowstorm. Photo credit: Margot Debyser, Woods Hole Oceanographic Institution. > High res figure
|
Finally, Revelle veered northeastward, leaving the Antarctic, sailing toward port across the fronts once more. Night returned, and with it, the last stations across the Chilean slope. Here, rosette casts brought Pacific Deep Water on deck, with the hope of capturing enhanced TEI flux signals from the Chilean margin. Then, one after another, glimpses of rain-sculpted land broke through the midmorning fog. Revelle purged her ballast, and we navigated the drowned Andean mountains of the Straits of Magellan.
In total, the GTC team had filtered 12,953 seawater samples from 59 sites, targeting 13 elements of interest for 21 laboratories.
Future Thoughts
At the time of writing, the GEOTRACES section-based science mission may be nearly complete, marking an exciting time of transition. Since the beginning of GEOTRACES in 2004, TEI data from over 130 international cruises have mapped biogeochemical parameters in every ocean basin, providing insightful visual representations of global micronutrient, contaminant, and isotopic data. The Standards & Intercalibration committee (S&I) rigorously assesses these datasets, submitted via the GEOTRACES DOoR portal (https://geotraces-portal.sedoo.fr/pi/), ensuring that only high-fidelity, intercalibrated measurements are consolidated into the publicly available GEOTRACES Intermediate Data Products (Mawji et al., 2015; Schlitzer et al., 2018), which are incorporated into free, digital education products like the eGEOTRACES Atlas (https://www.egeotraces.org). Despite this progress, many internal process hypotheses have only partially been addressed, leaving much work to be done and new questions to be answered (Tagliabue et al., 2016, 2023).
1. To complete internal process hypotheses, improve models, and better understand the impact of climate forcing on TEI cycles, the next iteration of the GEOTRACES program may need to integrate the original section-based mission with cruises planned specifically to answer regional process-driven hypotheses. These tend to favor relatively small collaborative endeavors (four to five principal investigators, or PIs), as was foreseen in the GEOTRACES Science Plan (SCOR Working Group, 2007). This transition will entail collaborative, hybrid-management, multi-PI proposals, with scientists seeking to investigate the nuance of unanswered internal processes, for example, the potential of submarine groundwater discharge as a significant source of TEIs to the open ocean (Howe et al., 2020).
2. Concomitantly, GEOTRACES scientists are looking to collaborate with the nascent BioGeoSCAPES program (https://biogeoscapes.org) toward bridging the gap between micronutrient biogeochemistry, proteomics, and phytoplankton ecology (see Saito et al., 2024, in this issue). BioGeoSCAPES unites scientists seeking to constrain the feedback mechanisms responsible for ocean metabolism. This would provide another critical dimension for interpreting TEI distributions, understanding microbial control on biogeochemical cycles, and better forecasting trends in biotically mediated carbon sequestration (Tagliabue, 2023).
The international GEOTRACES program aims to develop at least two more data products before the Ocean Decade ends in 2030 (Ryabinin et al., 2019). Then, a transition phase will be in order—one that gathers the leaders of the field, and our program, to begin focusing on single and multi-basin data synthesis. It would be beneficial to hold meetings modeled after Ocean Carbon & Biogeochemistry (OCB) scoping workshops (https://www.us-ocb.org/about/), which produce robust, coherent basin-wide stories pertaining to the cycling of trace elements. Most notably, these synthesis meetings provide strong leadership and prearranged agendas: preparation includes gauging interest, curating expertise, and supplying attendees with topics, questions, and objectives to stimulate focused discussions. Ideally, basin-scale data syntheses could catalyze publications that become foundational literature, consolidating all that is known and providing a framework for further investigations. These publications could then serve as a compass used by experts, lawmakers, and laypeople interested in the modern biogeochemistry of ocean basins. Regardless of the direction, so long as the culture and community of GEOTRACES is preserved, then the next iteration of the program will be undeniably prosperous.
Acknowledgments
I would like to thank Tim Conway, Jessica Fitzsimmons, Ben Twining, and countless others for their contributions to my career as a scientist. The international GEOTRACES program is possible in part thanks to the support from the US National Science Foundation (Grant OCE-2140395) to the Scientific Committee on Oceanic Research (SCOR).